Trypsin-hydrolyzed Corn Silk Proteins: Antioxidant Activities, in vitro Gastrointestinal and Thermal Stability, and Hematoprotective Effects
- DOI
- 10.2991/efood.k.200323.001How to use a DOI?
- Keywords
- Hematoprotection; lipid peroxidation; protein hydrolysate; simulated gastrointestinal digestion; stigma maydis; thermal stability
- Abstract
The aim of this study was to examine the antioxidant capacity of Trypsin-hydrolyzed corn silk proteins, specifically radical scavenging, ferric reducing and anti-lipid peroxidation activities, as well as stability after heating and simulated gastrointestinal digestion. Among the 1–5-h hydrolysates, the 1-h Trypsin hydrolysate (T1H) was the strongest. T1H exhibited stronger H2O2 [half maximal effective concentration (EC50) = 156.44 µg/mL] and superoxide (EC50 = 0.33 mg/mL) scavenging activities than glutathione, carnosine and N-acetylcysteine. Radical scavenging activities of T1H were heat-stable (25–100°C), although lost by 20–58% after gastrointestinal digestion. Ferric reducing activity of T1H was heat-stable, even enhanced by 20% after gastrointestinal digestion. Lecithin liposome assay found anti-lipid peroxidation activity of T1H resistant to gastrointestinal peptidases. By contrast, 100°C incubation reduced anti-lipid peroxidation activity of 0.5 mg/mL T1H to 3.5-fold lower than that of 25°C incubation. At 30 µg/mL, T1H (3% hemolysis) was stronger than glutathione (32% hemolysis) in protecting human red blood cells. T1H was 1.5-fold more potent than glutathione and carnosine in alleviating lipid peroxidation in the cells. Overall, T1H demonstrated strong, heat-stable radical scavenging activities, besides partial resistance to gastrointestinal peptidases. T1H was also hematoprotective. T1H is a promising antioxidant for the development of functional food ingredients and nutraceuticals.
- Copyright
- © 2020 International Association of Dietetic Nutrition and Safety. Publishing services by Atlantis Press International B.V.
- Open Access
- This is an open access article distributed under the CC BY-NC 4.0 license (http://creativecommons.org/licenses/by-nc/4.0/).
1. INTRODUCTION
Corn Silk (CS), the silky hair-like structures protruding from the tip of the ear of corn, is essentially the elongated stigma of the female flower of corn. Although often discarded as an agricultural waste or by-product of corn cultivation, CS has also been used as traditional remedies in different parts of the world for the treatment of kidney stones, diuretic, prostate disorders, urinary infections and obesity [1,2]. Much research has attributed the bioactivities and health-promoting potential of CS to its phenolic compounds, in particular flavonoids and terpenoids. Various CS-based teas and other commercial products with claimed health-promoting benefits are available in the market [1]. CS contains 9–22% protein content on a dry mass basis, depending on its stage of maturity and corn variety [3,4]. Thus CS may be a promising source of food proteins for the discovery of bioactive peptides.
Food-derived bioactive peptides have received growing attention from the international research community over the last two decades. Antioxidant peptides, both in the form of a protein hydrolysate as a mixture and in the form of pure individual peptides, are increasingly recognized as potent, natural alternatives to synthetic antioxidants for application as food additives. Such peptides are also potential candidates for future development of functional food ingredients and therapeutic agents [5–7]. To date, numerous antioxidant peptides and protein hydrolysates have been produced from food proteins of animal and plant origins [5,6]. However, bioactive peptide research on CS is practically non-existent, except for two studies which identified an antihypertensive peptide [8] and an anti-inflammatory peptide [9] from CS. The antioxidant capacity of CS protein hydrolysates and peptides is currently a gap in knowledge.
To fill the aforementioned gap of knowledge on the antioxidant potential of CS protein hydrolysates, this study was conducted to examine the antioxidant activities of Trypsin-hydrolyzed CS proteins by using a combination of in vitro chemical- and human red blood cell-based assays. Trypsin was used for proteolysis as it has been commonly and successfully used to produce food and non-food protein hydrolysates exhibiting antioxidant activities [10–14]. The use of multiple assays of different principles may reveal different modes of antioxidant action exerted by the CS protein hydrolysate. On the other hand, one concern regarding the effectiveness of antioxidant protein hydrolysates and peptides as food additives, health-promoting food ingredients, or as nutraceuticals is their stability towards heat treatment and gastrointestinal (GI) digestion. This concern is valid as heat treatment is common in food processing [15]; moreover, resistance to GI peptidases is key to the in vivo bioavailability and bioactivity of antioxidant peptides [16]. Thus, the objectives of this study were threefold: (i) to compare the antioxidant capacity of tryptic CS protein hydrolysates prepared by using different hydrolysis durations; (ii) to evaluate the stability of the most active hydrolysate against thermal treatments and in vitro gastrointestinal digestion; and (iii) to assess the ability of the most active hydrolysate to protect against cellular oxidative injury in a human red blood cell model.
2. MATERIALS AND METHODS
2.1. Corn Silk Sample and Reagents
Fresh corn silk was a gift from the owner of a local corn plantation, Mr Khuan Thean Ang. 2,2′-Azino-bis(3-ethylbenzthiazoline-6-sulfonic acid) (ABTS), thiobarbituric acid, and trichloroacetic acid were purchased from Merck (Germany). Pepsin and carnosine were purchased from Acros Organics (Belgium). Pancreatin (from porcine pancreas), glutathione (GSH), 2,2′-Azobis(2-methylpropionamidine) dihydrochloride (AAPH), and N-acetylcysteine (NAC) were purchased from Sigma-Aldrich (USA). Lecithin was purchased from Fisher Scientific (USA). O-phthalaldehyde (OPA) and Trypsin (from hog pancreas, 1539 U/mg) were purchased from Nacalai Tesque Inc. (Japan). Phosphate Buffered Saline (PBS) was purchased from Takara Bio Inc. (Japan). All reagents and solvents were of analytical grade.
2.2. Protein Isolation and Hydrolysis
Corn silk was oven-dried to constant weight at 50°C and pulverized with a blender. The resulting corn silk powder was defatted and subsequently used for the preparation of Corn Silk Protein Isolate (CSPI) via the pH-shift technique [17,18]. CSPI was lyophilized and stored at −20°C until used. Protein content of CSPI was measured with the Bradford’s assay [19], using a standard curve generated with 0.1–0.5 mg/mL bovine serum albumin. Flow diagram for the experimental design is shown in Figure 1.
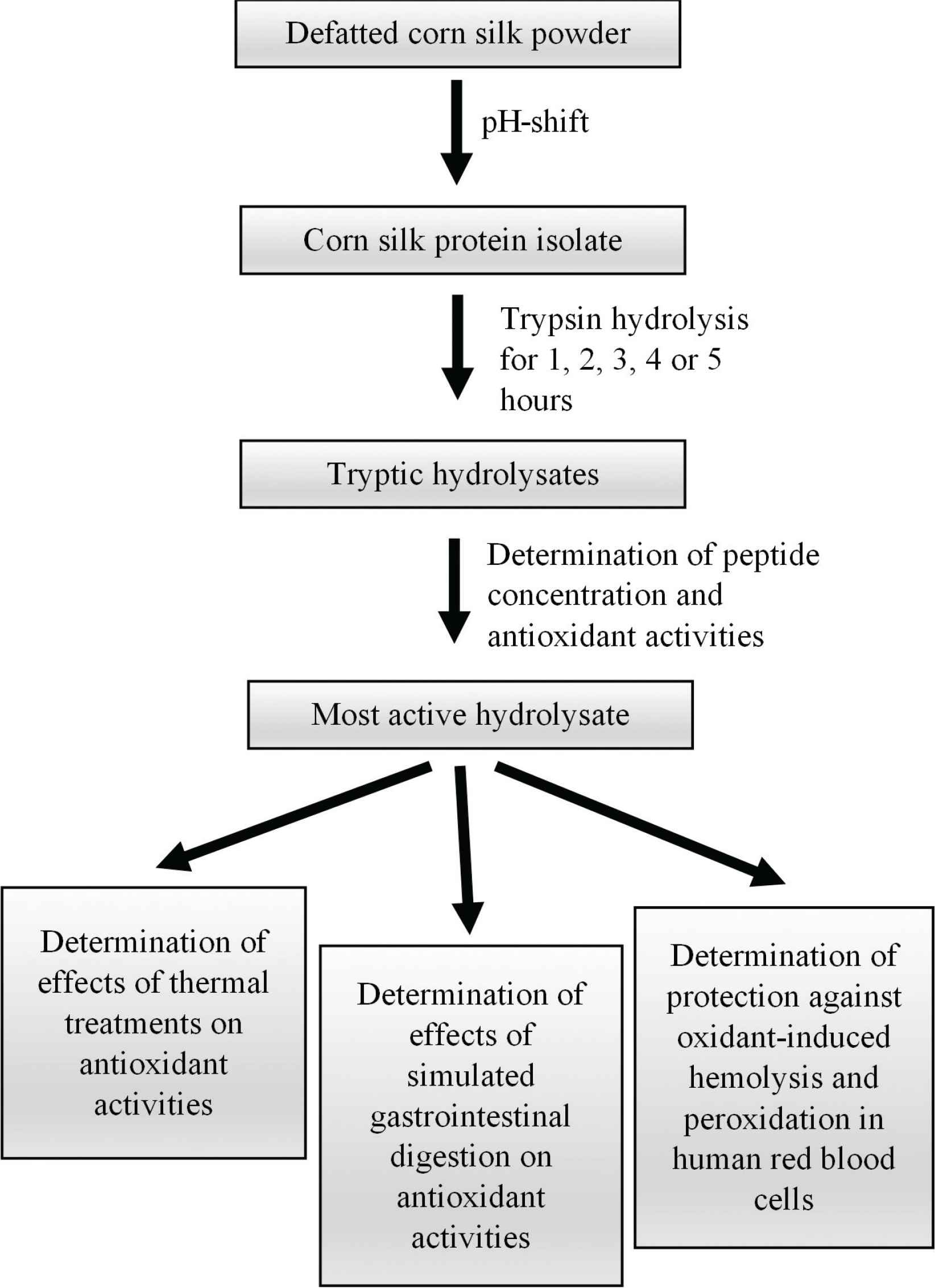
Flow diagram for experimental design.
CSPI was hydrolyzed with Trypsin for 1, 2, 3, 4, or 5 h under optimal temperature and pH conditions as previously described [20–22], with minor modifications. Briefly, CSPI was dissolved in 50 mM sodium phosphate buffer (pH 8) at a mass (g): volume (mL) ratio of 1:100. Protein concentration used for hydrolysate production was 6.76 mg proteins/mL. Trypsin was added at an enzyme (g): protein (g) ratio of 1:10. Trypsin concentration used for hydrolysate production was 1040 U/mL. Hydrolysis was carried out at 37°C and terminated by heating a hydrolysate aliquot in boiling water for 10 min. The heated hydrolysate was cooled on ice and then centrifuged at 10,000 × g and 4°C for 10 min to obtain the supernatant. The supernatant was tested for peptide concentration. Peptide content was determined by using the OPA assay as previously described [23], except that the standard curve was generated by using 0.1–0.5 mg/mL tryptone.
2.3. Determination of Radical Scavenging and Ferric Reducing Activities
2,2′-Azino-bis(3-ethylbenzthiazoline-6-sulfonic acid) radical cation (ABTS•+) scavenging activity was determined as described by Wong et al. [24], with modifications. Briefly, 20 µL of sample was mixed with 200 µL of ABTS working solution. After incubation in darkness for 20 min, the absorbance of the reaction mixture was measured at 734 nm. For comparison of the hydrolysates of different hours, the samples were tested at 10 µg peptide/mL. H2O2 scavenging activity was determined as previously described [25], with modifications. Briefly, 200 µL of sample was added to 600 µL of 40 mM H2O2 (in 50 mM PBS, pH 7.4). The mixture was allowed to stand for 10 min before its absorbance was recorded at 230 nm. To compare the hydrolysates of different hours, samples were tested at 62.5 µg peptide/mL. Superoxide scavenging activity was measured as described by Chai et al. [26], with modifications. A mixture of 160 µL of potassium phosphate buffer (100 mM, pH 7.4), 20 µL of sample, 20 µL of 0.78 mM nitro blue tetrazolium, 20 µL of 2.34 mM reduced nicotinamide adenine dinucleotide (NADH), and 10 µL of 60 µM phenazine methosulfate was incubated for 20 min in darkness. Thereafter, the absorbance of the mixture was read at 560 nm. For comparison of the hourly hydrolysates, samples were tested at 87 µg peptide/mL. For the aforementioned assays, EC50 (half-maximal effective concentration) was defined as the sample concentration required to achieve 50% activity.
Ferric reducing activity of the samples was determined with the Ferric Reducing Antioxidant Power (FRAP) assay [27], with modifications. Briefly, a mixture consisting of 50 µL of sample and 200 µL of FRAP reagent was incubated at 37°C for 5 min. Samples were tested at 1 mg peptide/mL, unless otherwise stated. Absorbance of the mixture was then read at 593 nm. FRAP values are presented in µM Fe2+ equivalents, calculated from a standard curve prepared with 0–0.40 mM FeSO4·7H2O.
2.4. Protection against Lipid Peroxidation in Lecithin Liposome Model
CSPI hydrolyzed with Trypsin for 1 h, designated as T1H, was tested for inhibitory activity against lipid peroxidation by using the lecithin liposome model system as described by Karnjanapratum and Benjakul [28], with modifications. Briefly, copper (II) acetate-induced lipid peroxidation in the model system was allowed to occur in the presence or absence of T1H (0.1 and 0.5 mg peptide/mL) in a temperature-controlled shaker (100 rpm) at 37°C. The reaction mixture consisted of 1 mL of T1H, 2 mL of sonicated lecithin suspension, and 15 µL of copper (II) acetate. For the blank, 1 mL of 50 mM sodium phosphate buffer (pH 8) was added in place of T1H. After 24 and 48 h of incubation, the levels of Thiobarbituric Acid Reactive Species (TBARS) in the mixtures were monitored at 532 and 600 nm [29,30]. TBARS levels were expressed as µM malondialdehyde (MDA) equivalents, calculated by using the molar extinction coefficient of MDA 1.56 × 105 /M/cm [31]. Percentage of inhibition of lipid peroxidation was computed by using the equation below. Ablank = absorbance of the blank (A532 − A600); Asample = absorbance of the sample (A532 − A600).
2.5. Effects of Thermal Treatments and Simulated GI Digestion
The stability of T1H against thermal treatments was determined as reported by Yarnpakdee et al. [32], with slight modifications. T1H was incubated in temperature-controlled water baths at 25, 65, 75, 85, and 100°C for 30 min. For ABTS•+, H2O2 and superoxide scavenging activity measurements, T1H concentration was tested at the EC50 of each parameter, namely 8, 31, and 65 µg peptide/mL. For FRAP assay, thermal-treated T1H was tested at 1 mg peptide/mL. For the determination of lipid peroxidation inhibitory activity by using the lecithin liposome model system, thermal-treated T1H was tested at 0.1 and 0.5 mg peptide/mL.
The stability of T1H against simulated GI digestion was assessed by using a two-stage digestion model (pepsin treatment for 1 h, followed by pancreatin treatment for 2 h) [33]. GI-digested T1H was lyophilized and its peptide content was determined with the OPA assay as described in Section 2.2. EC50 for the ABTS•+, H2O2 and superoxide scavenging activity of GI-digested T1H was determined. The FRAP value of GI-digested T1H was tested at 1 mg peptide/mL. To assess its ability to inhibit lipid peroxidation, GI-digested T1H was tested at 0.1 and 0.5 mg peptide/mL by using the lecithin liposome model system as described in Section 2.4.
2.6. Protection of Human Red Blood Cells from Oxidative Injury
The ability of T1H to protect against AAPH-induced oxidative hemolysis in normal human red blood cells was evaluated as described by Vinjamuri et al. [34], with modifications. Procedures involving human participants were performed in accordance with the ethical standards of the institutional and/or research committee and with Helsinki Declaration of 1975. Ethical approval was obtained from the UTAR Scientific and Ethical Review Committee (reference number: U/SERC/155/2018).
A mixture of 250 µL of 10% packed red blood cells and 500 µL of T1H was pre-incubated at 37°C for 60 min. Next, 500 µL of 200 mM AAPH (in PBS) was added to the mixture and incubation was continued. In two other sets of tubes, AAPH was substituted with PBS and 1% Triton X-100 (in PBS), respectively. T1H was tested at the final concentration of 10–30 µg peptide/mL. The aforementioned preparations were then subjected to 2-h incubation at 37°C. An aliquot of 200 µL of the incubated mixture was added with 600 µL of PBS. After centrifugation at 1500 × g for 10 min, absorbance of the supernatant was determined at 540 nm by using a microplate reader. Percentage of hemolysis was calculated by using the equation below. Asample, APBS and ATriton are absorbance readings corresponding to red blood cells treated with T1H and AAPH, with only PBS, and with only 1% Triton X-100, respectively.
The level of lipid peroxidation in the aforementioned AAPH-treated red blood cells was determined with the TBARS assay, as described in Section 2.4. For the blank, 50 mM sodium phosphate buffer (pH 8) was added in place of T1H. Percentage of inhibition of lipid peroxidation was computed by using the equation below. Ablank = absorbance of the blank (A532 − A600); Asample = absorbance of the sample (A532 − A600).
2.7. Data Analysis
Analyses were performed in triplicates and data are presented as mean ± Standard Errors (SE). Statistical analysis was performed by using the SAS software (version 9.4, SAS, USA). Data were analyzed by the one-way analysis of variance (ANOVA) test, followed by Fisher’s Least Significant Difference (LSD) test for the comparison of multiple means. Comparison of two means was performed by using Student’s t-test. p-value of <0.05 was considered statistically significant.
3. RESULTS AND DISCUSSION
In this study, proteins were isolated from corn silk, producing CSPI, which had 675.53 ± 13.34 mg proteins/g dry mass. CSPI was hydrolyzed with Trypsin for 1–5 h. Peptide concentrations of the hydrolysates increased from the 1st to the 4th hour (Table 1), implying that more and more peptides were released with time as CSPI was subjected to proteolysis. Over the 5 h of hydrolysis, the trends of changes in ABTS•+, H2O2, and superoxide scavenging activities and FRAP values were generally opposite of those of the peptide concentrations. Based on the four antioxidant assays, T1H had the greatest radical scavenging and ferric reducing activities. This implies that the antioxidant capacity of a protein hydrolysate is not necessarily directly proportional to its peptide abundance. As previously pointed out by Xiong et al. [35], enzymatic hydrolysis may release both antioxidant and non-antioxidant peptide fragments from the parent proteins. In this study, the stronger antioxidant effect of T1H may imply that it had a higher abundance of antioxidant peptides or peptides with stronger antioxidant capacity, relative to four other hourly hydrolysates. Thus subsequent analyses of the antioxidant capacity of tryptic CS protein hydrolysates in this study focused on T1H.
Duration (h) | Peptide concentration (mg/mL) | ABTS•+ scavenging activity (%) | H2O2 scavenging activity (%) | Superoxide scavenging activity (%) | FRAP (µM Fe2+ equivalents) |
---|---|---|---|---|---|
1 | 1.73 ± 0.14a | 66.55 ± 0.18a | 100.00 ± 0.00a | 62.32 ± 1.05a | 176 ± 3a |
2 | 2.56 ± 0.19b | 51.82 ± 1.82b | 53.73 ± 1.17b | 54.04 ± 0.90b | 155 ± 9b |
3 | 3.61 ± 0.18c | 41.66 ± 2.26c | 45.43 ± 2.30c | 45.08 ± 0.51c | 128 ± 8c |
4 | 3.77 ± 0.14c | 44.07 ± 1.53c | 38.40 ± 0.48d | 44.97 ± 0.72c | 122 ± 3c |
5 | 3.57 ± 0.14c | 44.18 ± 0.62c | 39.21 ± 0.93d | 48.60 ± 0.43d | 125 ± 1c |
Data are expressed as mean ± SE (n = 3). For each parameter, data labelled with different superscript letters are significantly different (p < 0.05) according to the Fisher’s LSD test.
Peptide concentration and antioxidant activities of CSPI hydrolyzed by Trypsin for 1–5 hours
The peptide content of T1H was 198.20 ± 1.80 mg/g dry mass. The yield of T1H from CSPI was 83% by mass. When expressed as µg or mg dry mass/mL, the EC50 values for the ABTS•+, H2O2, and superoxide scavenging activities of T1H were 42, 32, and 78% lower than those of CSPI (Table 2). FRAP value of T1H was 44% lower than that of CSPI. These observations suggest that T1H had stronger radical scavenging activities than CSPI, although the ferric reducing activity of CSPI was not improved by tryptic hydrolysis. There is a lack of reports in the literature concerning the antioxidant capacity of trypsin-hydrolyzed CS proteins. However, our observation of the enhancement of radical scavenging activities following trypsin hydrolysis is consistent with those reported in medicinal herb Ficus deltoidea [13], lupin [36], flaxseed [37] and porcine liver [38]. Enhanced ferric reducing activity following tryptic hydrolysis was found for flaxseed [37] and porcine liver [38] proteins, which is a contrast to our observation on CS proteins. The underlying reason for the discrepancy remains to be ascertained, but it may be in part due to the different protein sources used between the studies of others and ours.
Samples | ABTS•+ scavenging activity (EC50) (µg DM/mL) | H2O2 scavenging activity (EC50) (µg DM/mL) | Superoxide scavenging activity (EC50) (mg DM/mL) | FRAP (µM Fe2+ equivalents) |
---|---|---|---|---|
CSPI | 69.68 ± 0.25a | 228.64 ± 1.89a | 1.52 ± 0.01a | 314 ± 4a |
T1H | 40.73 ± 0.10b | 156.44 ± 0.88b | 0.33 ± 0.01b | 176 ± 3b |
GSH | 6.65 ± 0.07c | 2284.24 ± 4.80c | 6.35 ± 0.43c | 1144 ± 12c |
Carnosine | – | 1825.32 ± 12.44d | – | 5 ± 1d |
NAC | 1.28 ± 0.01d | 2970.32 ± 5.87e | 3.10 ± 0.01d | 1122 ± 34c |
Data are expressed as mean ± SE (n = 3). For each parameter, data labelled with different superscript letters are significantly different (p < 0.05) according to the Fisher’s LSD test. FRAP values of the samples were tested at 5 mg DM/mL. DM, dry mass.
Antioxidant activities of CSPI and T1H in comparison with positive controls
The antioxidant activities of T1H were also compared with those of reference antioxidants, namely GSH, carnosine and NAC. These well-established antioxidants were chosen for comparison with T1H as they are either peptides (GSH and carnosine) or derivative of an amino acid (NAC), thus making them more appropriate to our study than non-peptide- or non-amino-acid-based antioxidants, such as quercetin and butylated hydroxytoluene. Based on the H2O2 scavenging assay, the EC50 values of GSH, carnosine and NAC were at least 12-fold higher than that of T1H. Based on the superoxide scavenging assay, the EC50 values of GSH and NAC were 19-fold and ninefold higher than that of T1H, respectively. EC50 of carnosine was not calculated owing to its negligible superoxide scavenging activity. Based on the ABTS•+ scavenging assay, the EC50 value of T1H was sixfold and 32-fold higher than those of GSH and NAC, respectively. For this assay, the EC50 of carnosine was also not calculated due to its very low activity. The FRAP value of T1H was higher than that of carnosine, but lower than those of GSH and NAC. Altogether, our results show that T1H was a weak ABTS•+ scavenger and reductant. However, T1H was a more powerful H2O2 and superoxide scavenger than GSH, carnosine, and NAC. This finding is of interest for two reasons: (i) H2O2 and superoxide radicals are biological relevant, and (ii) crude or unpurified protein hydrolysates reported by other studies were often less potent than well-established antioxidants. For example, in marked contrast with T1H, thermolysin-hydrolyzed pea seed proteins was about 18-fold weaker than glutathione in scavenging superoxide radicals [39]. The superoxide scavenging activities of trypsin, alcalase and pepsin hydrolysates of the Bambara groundnut were at least fivefold weaker than that of glutathione [40]. Furthermore, the superoxide scavenging activity of hemp seed meal consecutively hydrolyzed with pepsin and pancreatin was also drastically lower than that of glutathione [41]. Hence, considering the promising antioxidant potential of T1H, we further characterized the ability of T1H to protect against lipid peroxidation by using the lecithin liposome model.
As shown in Figure 2A, when incubated over 48 h in the absence of T1H, the level of lipid peroxidation rose with time. The inclusion of T1H reduced the level of lipid peroxidation in the lecithin liposome model in a concentration-depend manner. Nevertheless, T1H did not reverse the time-dependent rise in lipid peroxidation in the liposome model. A similar time-dependent increase in lipid peroxidation in the same model was also reported by Karnjanapratum and Benjakul [28] when investigating the antioxidant effect of gelatin hydrolysates derived from unicorn leatherjacket skin. The antioxidant activity of food protein hydrolysates and peptides, particularly in protecting against lipid peroxidation, has been associated with the presence of hydrophobic residues, such as alanine, leucine, and proline [6]. Hence the ability of T1H to repress lipid peroxidation implies the presence of hydrophobic residue-containing peptides in the hydrolysate. When compared with reference antioxidants, T1H was as effective as carnosine. However, its inhibition of lipid peroxidation was 2.7–3.8-folds weaker than GSH and NAC (Figure 2B). Future work can further confirm the effect of T1H in inhibiting lipid peroxidation by substituting the Cu2+ catalyst with alternatives such as Fe2+/ascorbate, H2O2 and AAPH. Evaluation of T1H for copper and iron chelating activities may also provide a clue as to whether the lipid peroxidation inhibitory activity of T1H is primarily due to its metal chelating action.
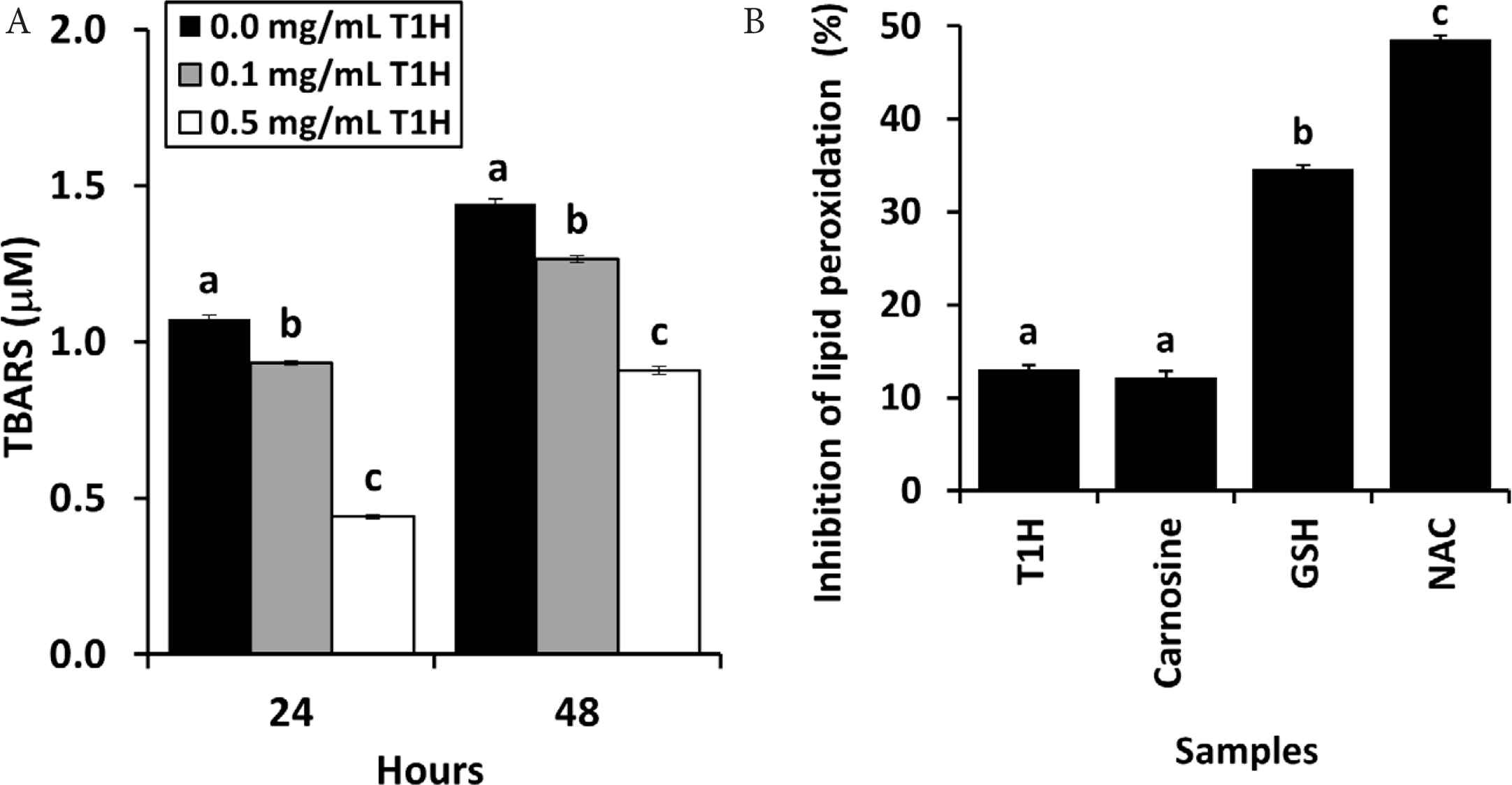
(A) TBARS levels of lecithin liposome model supplemented with T1H and incubated for 24 and 48 h. (B) Ability of 0.1 mg peptide/mL T1H to inhibit lipid oxidation in the 24-h lecithin liposome model, in comparison with carnosine, GSH and NAC. Data are expressed as mean ± SE (n = 3). Data labelled with different letters are significantly different (p < 0.05) according to the Fisher’s LSD test.
Food protein hydrolysates are recognized for their potential applications as natural antioxidants that can be incorporated in food products [42,43]. T1H possessed different degrees of free radical scavenging, ferric reducing and lipid peroxidation inhibitory activities. Thus, it may potentially be developed into an antioxidant additive for food products. Thermal treatment is one of the main treatments involved in food processing [15]. A suitable natural antioxidant additive should ideally be heat-stable. Thus, we subjected T1H to thermal treatments of up to 100°C and assessed their effects on the antioxidant capacity of T1H. Overall, the peptide concentration of T1H was stable. The 100°C-treated T1H sample still maintained about 90% of the peptide concentration of 25°C-treated T1H (Figure 3A). T1H was also assessed for ABTS•+, H2O2, and superoxide scavenging activities at its EC50 values. For these assessments, the activities of T1H were at about the 50% level. Generally, there was no statistically significant difference between the different temperature treatments (Figure 3B–3E). Our finding is in accordance with the observation of heat-stable ABTS•+ scavenging activity in Nile tilapia protein hydrolysate [32], semen cassiae protein hydrolysate [23], mung bean meal-derived antioxidant peptides [44] and blue-spotted stingray-derived peptides [24]. In this study, a temperature-dependent decrease in the inhibition of lipid peroxidation, as assessed by using the lecithin liposome model, was detected (Figure 3F). This suggests that the peptides in T1H that protected against lecithin peroxidation were not completely heat-stable. Heat treatments can cause degradation or aggregation of some antioxidant peptides, dampening their activity [32,45]. In addition, instability of antioxidant peptides under high temperatures may also be ascribed to changes in their secondary structure [46]. Thus the temperature-dependent decrease in the lipid peroxidation inhibitory activity of T1H was possibly attributable to heat-induced peptide degradation/aggregation and/or instability of peptide secondary structure. Interestingly, in contrast to the inhibition of lipid peroxidation, the radical scavenging and reducing activities of T1H were heat-stable. This observation suggests that peptides differing in thermal stability may have accounted for the radical scavenging and reducing activities and for the lipid peroxidation inhibitory activity of T1H. Overall, considering the thermal stability of T1H as a free radical scavenger and reductant, the possibility of its use as antioxidant additive may not be completely ruled out. Moreover, its potential application as antioxidant additive under low temperatures in frozen food products can also be explored in future.
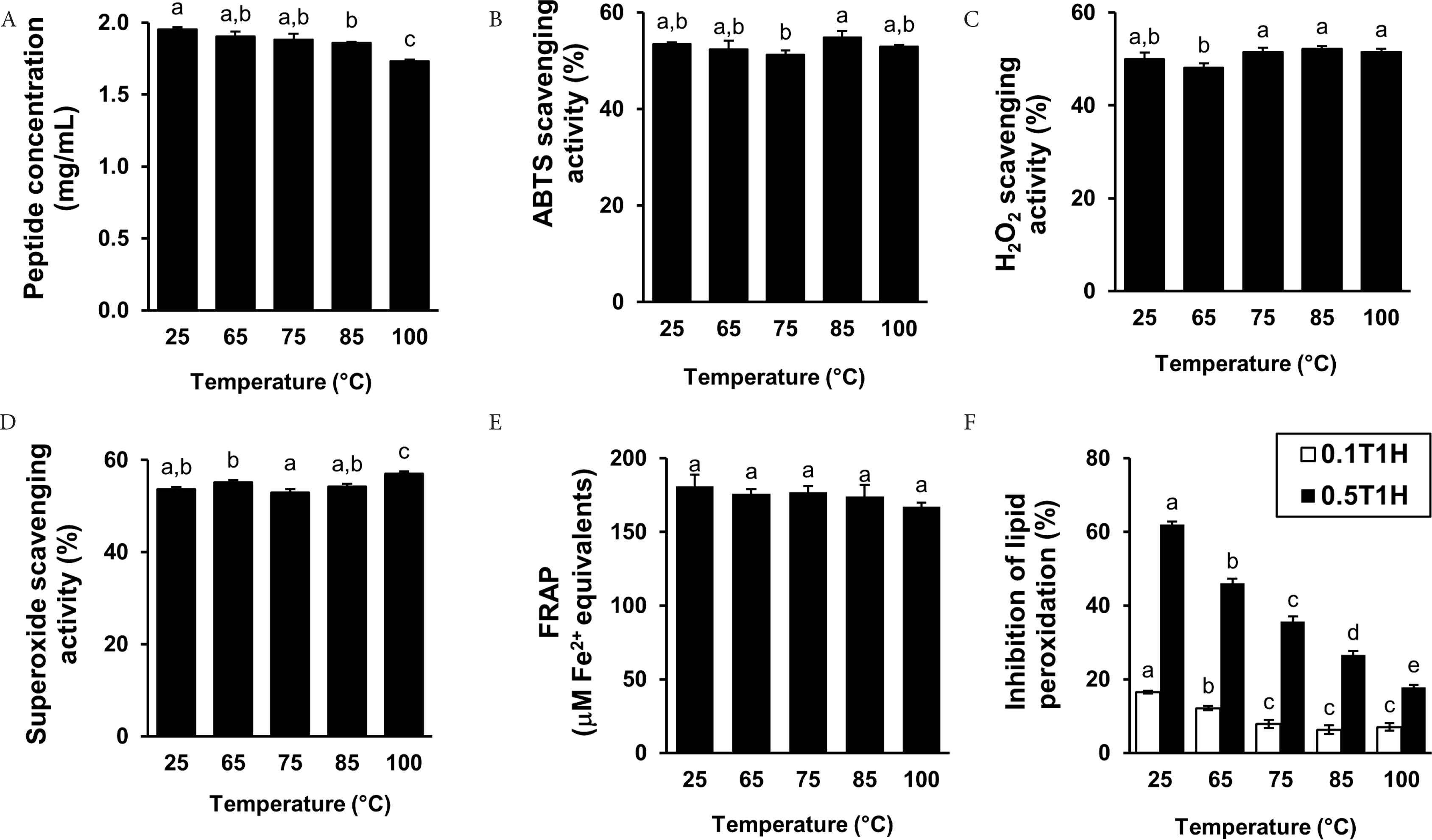
Peptide concentration (A), ABTS•+ scavenging activity (B), H2O2 scavenging activity (C), superoxide scavenging activity (D), FRAP (E), and lipid peroxidation inhibitory activity (F) of T1H pre-incubated under different temperatures. For (F), 0.1T1H and 0.5T1H represent 0.1 and 0.5 mg peptide/mL T1H, respectively. Data are expressed as mean ± SE (n = 3). Data labelled with different letters are significantly different (p < 0.05) according to the Fisher’s LSD test.
Besides incorporating T1H into food products as an antioxidant additive, another potential application of T1H is using it as a health-promoting food ingredient. However, one concern that often arises regarding peptides and protein hydrolysates is their bioavailability upon oral consumption. Simulated GI digestion is useful as an early-stage screening tool to predict whether the bioactivity of food protein hydrolysates and peptides would be retained following in vivo GI digestion [47]. In this study, a mild decline of 17% in the peptide content of T1H was detected after simulated GI digestion (Figure 4A). Meanwhile, the radical scavenging capacity of simulated GI-digested T1H showed concentration-dependent trends (Data not shown). Although T1H retained its radical scavenging activities after simulated GI digestion, the 20–58% increase in EC50 values indicates weakening of the activities to different extents (Figure 4B–4D). The decline in radical scavenging activity may also be ascribed to the degradation of some GI-susceptible antioxidant peptides in T1H, producing less- or non-active fragments, even free amino acids. T1H was not as superior as the semen cassiae [23] and loach [48] protein hydrolysates which maintained their ABTS•+ scavenging activities after simulated GI digestion. Interestingly, there is a mild improvement by 20% in the ferric reducing activity of T1H, as indicated by the higher FRAP value resulting from simulated GI digestion (Figure 4E). Our finding agrees with the report of enhanced reducing power of loach protein hydrolysate after simulated GI digestion [48]. Moreover, the improved reducing power of the GI digest of T1H implies that the digested T1H may contain peptides that were more effective hydrogen or electron donors when compared with undigested T1H [48].
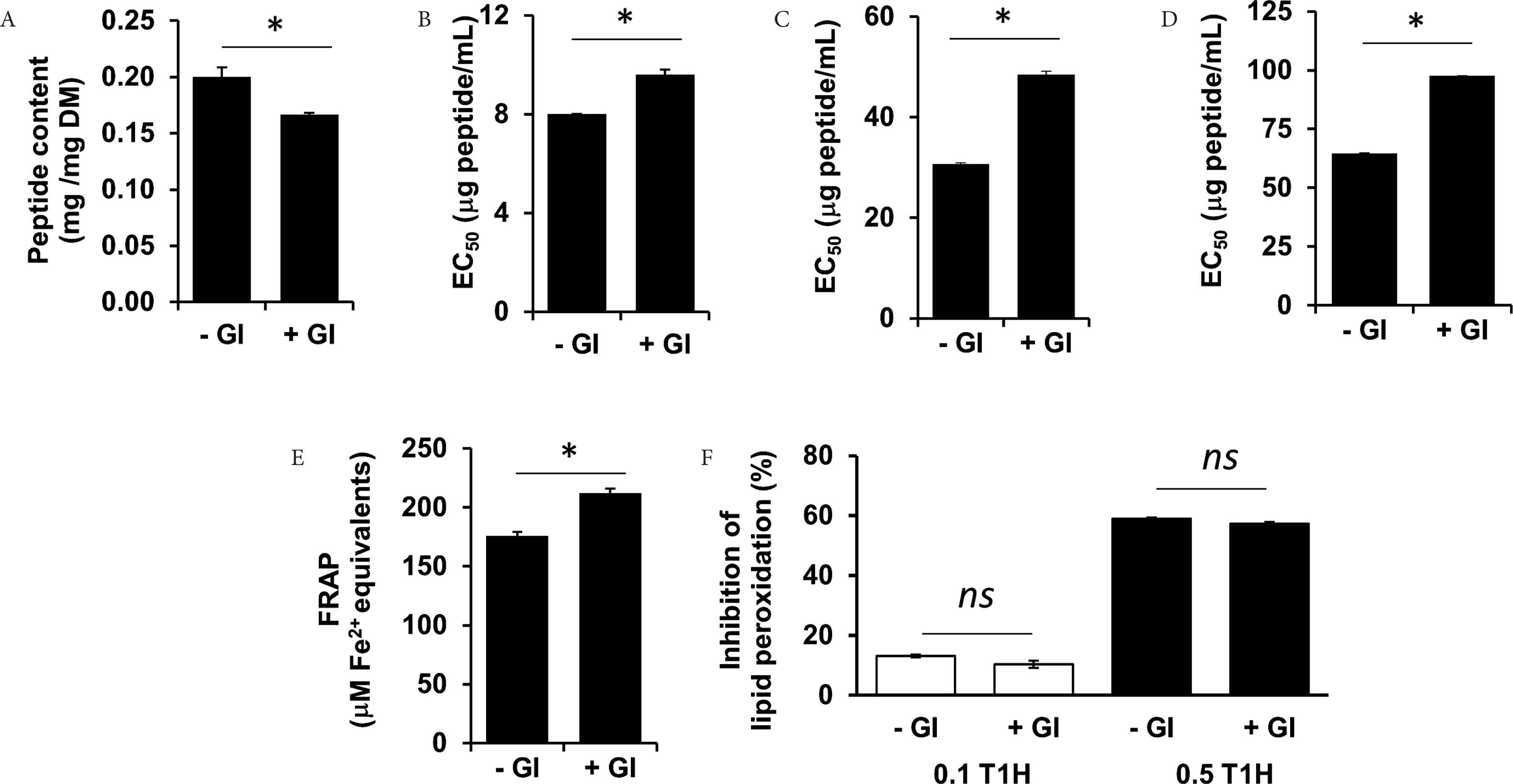
Peptide content (A), ABTS•+ scavenging activity (B), H2O2 scavenging activity (C), superoxide scavenging activity (D), FRAP (E) and lipid peroxidation inhibitory activity (F) of T1H before and after simulated GI digestion. For (F), 0.1T1H and 0.5T1H represent 0.1 and 0.5 mg peptide/mL T1H, respectively. Asterisks (*) denote significant differences (p < 0.05) between undigested (−GI) and GI-digested (+GI) T1H, according to Student’s t-test. ns denotes lack of statistical significance. Data are expressed as mean ± SE (n = 3).
On the other hand, the lipid peroxidation inhibitory activity of T1H was stable to simulated GI digestion (Figure 4F). The simulated GI-digested T1H also showed concentration-dependent inhibition of lipid peroxidation. The lipid peroxidation inhibitory activity of T1H is superior in stability to that of the loach protein hydrolysate, which decreased by about threefolds after simulated GI digestion [48]. Such stability may be attributed to the GI resistance of those peptides in T1H that were responsible for the lipid peroxidation activity detected. Certain antioxidant peptides, for example, those containing proline, are often resistant to the action of GI digestive enzymes [24,49]. Whether such peptides are found in T1H remains to be determined in future. Considered as a whole, our results point to partial resistance of T1H to GI digestion and thus T1H may be potentially bioavailable when consumed. Future experiments may use the Caco-2 cell monolayer, an intestinal absorption model [50], to further characterize the potential bioavailability of T1H.
Granato et al. [51] pointed out that the biological relevance of an antioxidant cannot be adequately or accurately characterized by applying only chemical-based antioxidant assays. Hence, in this study, using human red blood cells as the cellular model, we evaluated whether T1H protected against AAPH-induced oxidative injury, manifested as hemolysis and cellular lipid peroxidation. As shown in Figure 5A, T1H, GSH and carnosine all inhibited oxidative hemolysis in a concentration-dependent manner. When AAPH-stressed red blood cells were treated with 30 µg/mL T1H or carnosine, hemolysis levels dropped by at least 25-fold to the negligible 3% and 2%, respectively. In other words, 30 µg/mL of T1H almost eliminated hemolysis in the AAPH-treated red blood cells. By contrast, 30 µg/mL of GSH only reduced hemolysis percentage from 77% to 32%, a decline of 2.4-fold. These observations suggest that T1H was as effective as carnosine, but more potent than GSH, in suppressing oxidative hemolysis. Figure 5B shows that T1H, GSH and carnosine inhibited lipid peroxidation in the AAPH-treated red blood cells in a concentration-dependent manner. At 30 µg/mL, the lipid peroxidation inhibitory activity of T1H was 1.5- and 1.4-fold higher than those of GSH and carnosine. Our finding highlights that T1H could protect human red blood cells from oxidative injury more effectively than well-established antioxidants GSH and carnosine. Interestingly, T1H is comparable to blue-spotted stingray-derived peptides that were also more potent than carnosine as inhibitor of lipid peroxidation in the red blood cells [24].
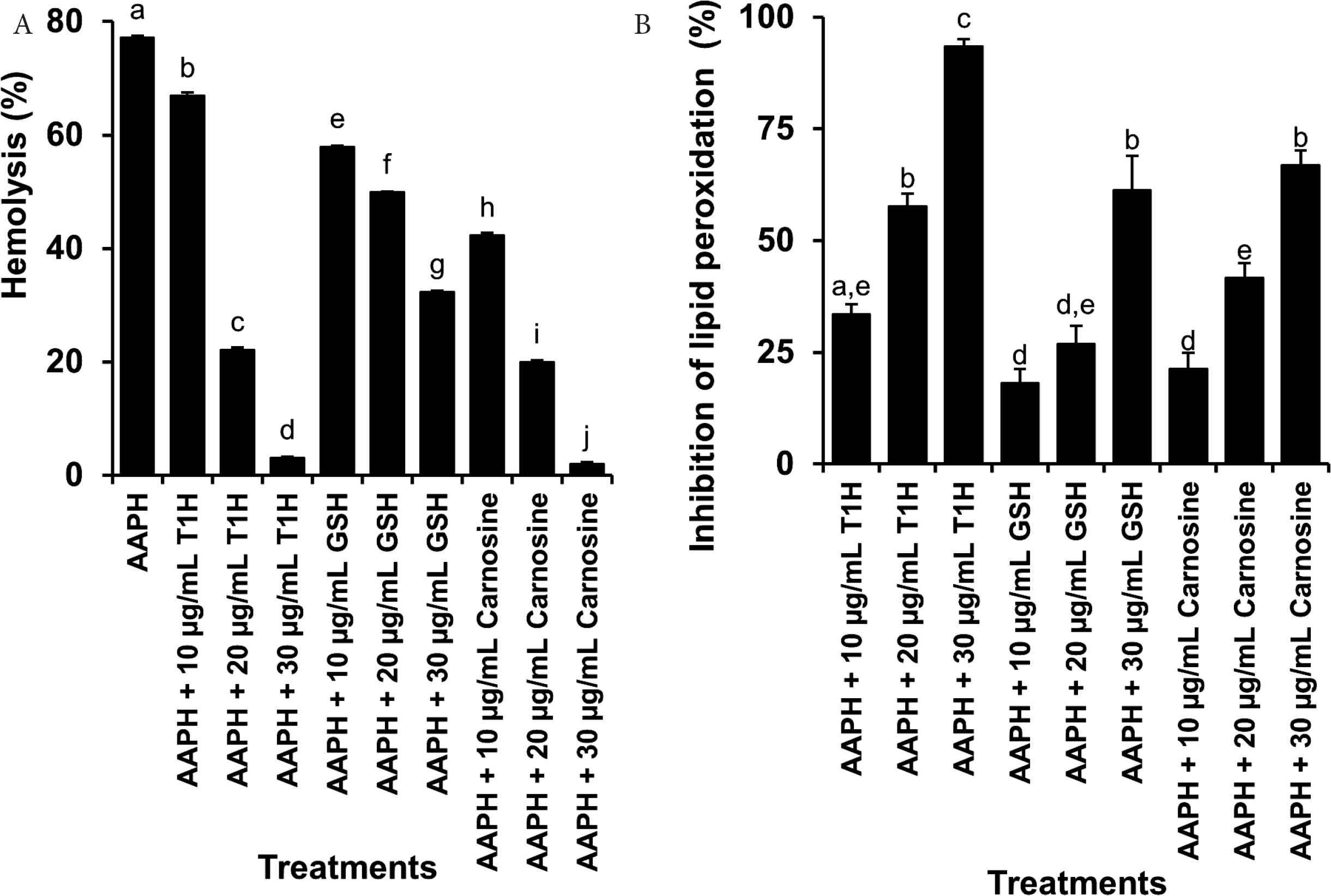
Ability of T1H, GSH and carnosine to inhibit AAPH-induced oxidative hemolysis (A) and lipid peroxidation (B) in red blood cells. Data are expressed as mean ± SE (n = 3). Data labelled with different letters are significantly different (p < 0.05) according to the Fisher’s LSD test.
Our red blood cell assays (Figure 5) confirm our results from the lecithin liposome assay (Figure 2) that T1H indeed possessed lipid peroxidation inhibitory activity. However, unlike the outcome of our lecithin liposome experiment, T1H was consistently stronger than GSH and carnosine in protecting red blood cells against AAPH-induced oxidative injury. Such a discrepancy may have risen due to the different mechanisms by which lipid peroxidation was induced in the lecithin liposome and the red blood cell models. Hence, our results suggest that the use of different models is desirable to allow a more complete characterization of the lipid peroxidation inhibitory effects of protein hydrolysates and peptides. Future research may consider testing the lipid peroxidation inhibitory ability of T1H with other oxidant-challenged cellular and animal models, such as H2O2-treated red blood cells [24], H2O2-treated HepG2 cells [52], and mice administered with tert-butyl hydroperoxide intraperitoneally, in which liver oxidative damage can be monitored [53].
4. CONCLUSION
In this study, we demonstrated for the first time the antioxidant capacity of CS protein hydrolysates. Comparison of the antioxidant activities of the five hourly hydrolysates found T1H to be the most active. T1H possessed strong H2O2 and superoxide scavenging activities. Overall, the radical scavenging activities of T1H were heat-stable, but were partially lost after simulated GI digestion. The lipid peroxidation inhibitory activity of T1H was stable after simulated GI digestion, but not after heat treatment. Notably, T1H also exhibited strong protection against lipid peroxidation in the oxidant-treated human red blood cells. Taken together, T1H is a promising candidate for the development of functional food or beverage ingredients and nutraceuticals. Hence future research to better characterize the cellular and in vivo effects of T1H, particularly its intestinal absorption and metabolism as well as safety for consumption, is warranted. Future research to purify the specific peptides that were responsible for the antioxidant effects of T1H will also facilitate a better understanding of the modes of action of the hydrolysate. Overall, our findings suggest that CS is a promising source of antioxidant peptides, which deserves more attention from researchers in future.
CONFLICTS OF INTEREST
The authors declare they have no conflicts of interest.
AUTHORS’ CONTRIBUTION
TTC, LKT and FCW contributed in conceptualization. YHL, JMN, SYA and KG contributed in literature search, experimental data collection and analyses. TTC and LKT contributed in study design. TTC and FCW contributed in manuscript writing and editing.
Footnotes
REFERENCES
Cite this article
TY - JOUR AU - Tsun-Thai Chai AU - Shin-Yii Ang AU - Kervine Goh AU - You-Han Lee AU - Jia-Min Ngoo AU - Lai-Kuan Teh AU - Fai-Chu Wong PY - 2020 DA - 2020/03/27 TI - Trypsin-hydrolyzed Corn Silk Proteins: Antioxidant Activities, in vitro Gastrointestinal and Thermal Stability, and Hematoprotective Effects JO - eFood SP - 156 EP - 164 VL - 1 IS - 2 SN - 2666-3066 UR - https://doi.org/10.2991/efood.k.200323.001 DO - 10.2991/efood.k.200323.001 ID - Chai2020 ER -