Molecular mechanisms of arterial calcification
- DOI
- 10.1016/j.artres.2009.10.001How to use a DOI?
- Copyright
- © 2009 Association for Research into Arterial Structure and Physiology. Published by Elsevier B.V. All rights reserved.
- Open Access
- This is an open access article distributed under the CC BY-NC license.
Vascular calcification is a pathological process that occurs at two main sites in the artery wall: the intima and the media. Intimal calcification is found in atherosclerotic plaques and it is closely associated with vascular smooth muscle cells (VSMCs), macrophages and lipid. Medial calcification is common in diabetes, kidney disease and aging and often appears linearly in association with elastin fibres and VSMCs. Intimal calcification has been linked with atherosclerotic plaque rupture1 and medial calcification is also detrimental as it causes arterial stiffness which leads to an increase in cardiac work and pulse pressure, which is a highly significant predictor of myocardial infarction.2
Evidence for regulation of calcification
Calcification was once thought to be a passive, degenerative consequence of aging but it is now recognised as a regulated process with similarities to bone formation. Although fairly uncommon, true bone formation has been detected in both the intima and media of human arteries. The calcification that occurs in the vessel wall and in bone is similar to hydroxyapatite, Ca10(PO4)6(OH)2, with several variants reported such as octacalcium phosphate, carbonate-substituted hydroxyapatite, and amorphous calcium phosphate.3–5 In bone, nucleation of calcium crystals occurs in matrix vesicles which are nanoparticulate, membrane-bound structures released from osteoblasts and chondrocytes. Similar structures released from VSMCs have been detected in human calcified arteries and from cultured cells.4,6 VSMCs have also been shown to undergo osteochondrocytic differentiation, expressing many of the factors normally associated with bone formation, such as Cbfa1/Runx2 and alkaline phosphatase.7 VSMCs are therefore likely to play a major role in regulating calcification in the diseased vessel wall.
The concentration of calcium and phosphate ions in extracellular fluids is sufficiently high to cause the precipitation of calcium in tissues, however, this does not occur in health, which implies that calcification is actively inhibited. Studies in mouse knockout models have convincingly shown that calcification is under the control of several calcification inhibitors. These include: matrix gla protein (MGP); a protein previously associated with cartilage and bone; osteoprotegerin, a member of the TNF superfamily which regulates osteoclast differentiation; Klotho, a protein associated with aging; fibroblast growth factor-23 (FGF23), a circulating factor released from bone in response to high phosphate levels; fetuin, a circulating protein which binds hydroxyapatite and also a acts as an opsonin (facilitates phagocytosis); carbonic anhydrase II, an enzyme whose role is to regulate pH and Smad6, an intracellular mediator of bone morphogenetic protein signalling. The most striking of these models is the MGP knockout mouse where the large elastic arteries calcify extensively leading to death from arterial rupture within 2 months of birth.8 MGP contains 5 residues of an uncommon amino acid, γ-carboxyglutamic acid (Gla), formed by a vitamin K-dependent modification of specific glutamic acid residues. The Gla residues confer calcium and hydroxyapatite-binding properties, which may explain their inhibitory effects on calcification. Interestingly, the cells present in the arteries of the MGP knockout mice resemble chondrocytes rather than VSMCs which implies that MGP is also required to maintain the VSMC phenotype. This effect may be achieved via its binding to and inactivation of bone morphogenetic protein 2 (BMP-2), a potent inducer of calcification and bone formation.9
In man, an extremely rare disorder, Keutel Syndrome, characterised by abnormal cartilage calcification, is due to mutations in the MGP gene that predict truncated forms of MGP.10 These patients also develop vascular calcification.11 In addition, it is now know that ‘idiopathic calcification of infancy’ is due to mutations in ectonucleotide pyrophosphatasephosphodiesterase 1 (NPP1),12 a plasma membrane enzyme involved in pyrophosphate metabolism. Together, these conditions suggest a genetic component to the regulation of arterial calcification in man.
Effects of MGP on human VSMC calcification
Our studies have shown that MGP is expressed by VSMCs and is up-regulated at sites of calcification. Using an in vitro model of calcification where VSMCs spontaneously form multicellular nodules which calcify, MGP mRNA was highly up-regulated in the calcified nodules, compared with monolayer cultures.13 Treating the VSMC nodular cultures with vitamin K to encourage γ-carboxylation of MGP inhibited calcification, whereas treatment with the vitamin K-antagonist warfarin stimulated calcification, suggesting that γ-carboxylation of MGP is important in maintaining its anti-calcifying activity. To specifically test the effects of MGP on VSMC calcification, we used full-length synthetic MGP and MGP-derived peptides representing various domains in MGP. Full length MGP, the γ-carboxylated motif (Gla) (aa 35–54) and the phosphorylated-serine motif (aa 3–15) inhibited calcification.14 This revealed that both γ-glutamyl carboxylation and serine phosphorylation of MGP contribute to its function as a calcification inhibitor. Similarly, the calcification inhibitor osteopontin has been reported to inhibit calcification only in its phosphorylated form. MGP is thought to be expressed where calcium levels are high and we found that MGP protein expression was up-regulated in VSMC cultures exposed to twice the physiological level of calcium ions (3.6mM). However, the matrix vesicles isolated from these high calcium cultures contained less MGP protein than the matrix vesicles isolated under normal conditions. This may help to explain why calcification occurs where calcium ion levels are raised.
Role of cell death in calcification
Analysis of sections of calcified multicellular VSMC nodules showed that they contained fewer cells as they progressed with time in culture and fewer cells correlated with a higher degree of calcification. Confocal microcroscopy and nuclear staining techniques revealed that apoptosis occurred within the nodules. Stimulation of apoptosis in the VSMC nodules with anti-Fas antibodies increased calcification and inhibition of apoptosis with ZVAD (a caspase inhibitor) inhibited calcification, suggesting a role for apoptosis in stimulating calcification.15 In a similar manner to chondrocyte apoptotic bodies, we found that VSMC apoptotic bodies could concentrate and crystallise calcium in vitro. Under physiological conditions, apoptotic bodies are rapidly phagocytosed by adjacent phagocytic cells, including VSMCs. However, in the diseased vessel wall there may be VSMC loss, a reduced level of opsonins such as fetuin, and in atherosclerosis the presence of modified lipids reduces apoptotic body phagocytosis thereby enhancing calcification.16 A lack of phagocytosis of apoptotic cells would also lead to secondary necrosis where calcium and phosphate ions released from necrotic cells creates an environment favouring calcification. These in vitro observations have recently been demonstrated in vivo: VSMC apoptosis was reported to promote atherosclerosis and calcification in a mouse model,17 and apoptosis was shown to occur prior to calcification in arteries from dialysis patients.18 Therefore, rather than simply a degenerative mechanism, cell death via apoptosis and the release of apoptotic bodies that can form a nidus for calcification appear to play a major role in initiating calcification in vivo.
Biological activity of calcium phosphate crystals
Some recent studies have suggested that the very small, nano- and microparticulate calcium phosphate crystals that appear as ‘spotty’ or ‘speckled’ deposits in atherosclerosis cause plaque stress and instability.19–21 Studies in macrophages and synovial fibroblasts suggest that these small calcium phosphate crystals are pro-inflammatory and damaging.22,23 Our studies found that calcium deposits isolated from human arteries and synthetic hydroxyapatite caused human VSMC death.24 Crystals of less than 1μm in diameter caused these effects, with larger particles having no effect on cell viability, possibly due to a lack of phagocytosis of larger particles. Of the different types of synthetic crystals analysed, amorphous calcium phosphate had the greatest effect in reducing cell viability and the human crystal preparations were less potent than the synthetic preparations. This is likely due to the presence of associated proteins in the human calcified extracts which may have anticalcifying and antiapoptotic activities, such as fetuin.
The mechanism involved in the VSMC death involved a rapid rise in intracellular calcium ion levels. In approximately half of the cells treated with calcium deposits, the calcium ion elevations were sustained and did not return to baseline and these cells went on to die. The calcium levels in the remaining cells returned to baseline and these cells survived. Why only half of the cells treated with calcium deposits died is intriguing and may be due to the well-known heterogeneous nature of VSMCs. The exact mechanism of induction of VSMC death by the crystals is not known but in the presence of the lysosomal proton pump inhibitor, bafilomycin A1, the magnitude of the intracellular calcium ion concentration rises were smaller in response to calcium phosphate crystals and importantly, the cells remained viable. This suggests that the crystals are engulfed by VSMCs, processed to the lysosomes where the acidic environment dissolves the crystals and free calcium and phosphate ions are released into the cell. We can speculate that in some cells this extra calcium is effectively removed from the cell via membrane pumps or sequestered by intracelluar calcium stores, while calcium overload occurs in the remainder which die. The exact mechanisms involved in the crystal-induced cell death will be the focus of our future studies. These studies are important because VSMCs have a protective role in plaque rupture and the microparticulate calcium phosphate crystals could destabilise plaques by causing VSMC death.
Balance between inhibitors and initiators of calcification
In the diseased vessel wall there may be several factors that contribute to the initiation or stimulation of calcification (see Fig. 1). These include: apoptosis/necrosis, apoptotic bodies, matrix vesicles, cholesterol, modified lipids, calcium and phosphate ions, oxidative stress, tumour necrosis factor α, transforming growth factor β, interleukin-1 and 6, BMP-2, bone sialoprotein, alkaline phosphatase, elastin fragmentation and some drugs such as warfarin. Particularly in atherosclerosis where there may be several cell types present, there will be complex cellular interactions as well as physico-chemical interactions determining whether calcification is initiated and allowed to progress. Thus, the resulting calcification will be determined by the balance between factors with opposing actions in the complex diseased vessel wall environment.
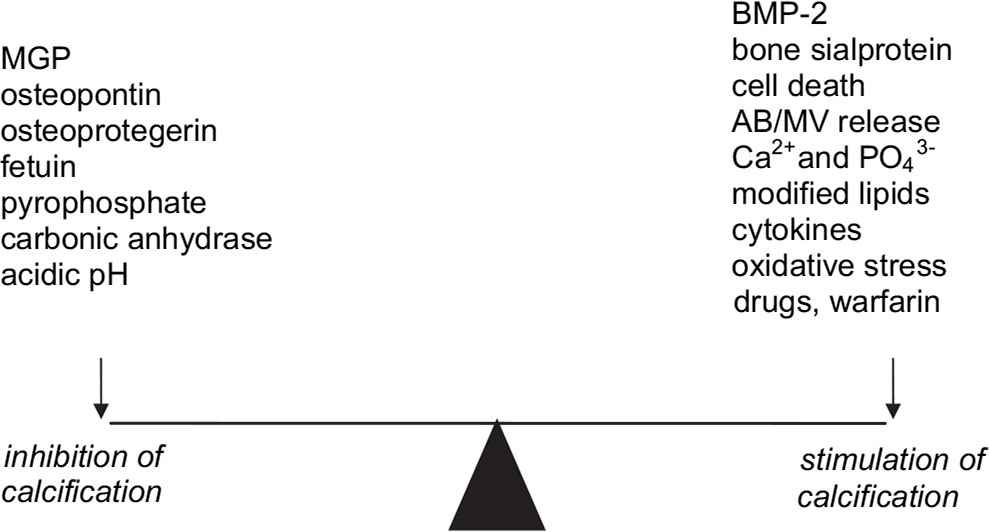
Balance between some of the inhibitors and triggers of calcifiction in the artery wall. AB=apoptotic body, MV=matrix vesicle.
Our most recent studies suggest that when VSMC death occurs in the vessel wall, apoptotic bodies and matrix vesicles create a nidus for calcification to occur. These nano- and microcalcific particles are engulfed by neighbouring VSMCs which causes further VSMC death, leading to a positive feedback cycle of death and calcification. In the diseased vessel wall, a lack of inhibitors of calcification and phagocytosis would allow this process to progress unchecked. Strategies to prevent VSMC death and enhance production and activity of calcification inhibitors such as MGP and fetuin may provide some therapeutic targets.
References
Cite this article
TY - JOUR AU - Diane Proudfoot PY - 2009 DA - 2009/10/29 TI - Molecular mechanisms of arterial calcification JO - Artery Research SP - 128 EP - 131 VL - 3 IS - 4 SN - 1876-4401 UR - https://doi.org/10.1016/j.artres.2009.10.001 DO - 10.1016/j.artres.2009.10.001 ID - Proudfoot2009 ER -