Magnetic resonance imaging in vascular biology☆
Grant support: M. Voehringer is supported by a research scholarship of Robert-Bosch Foundation, Germany, and is a trainee in the strategic training programme TORCH of University of Calgary and University of Alberta.
- DOI
- 10.1016/j.artres.2008.01.002How to use a DOI?
- Keywords
- Magnetic resonance imaging; Atherosclerosis; Imaging; Vessels
- Abstract
Symptoms are only the tip of the iceberg in atherosclerotic disease. Beneath the surface are multiple patho-physiological processes taking place in and around the vessel wall. The increasing knowledge in the field of vascular biology also reveals new imaging targets as biological markers of the disease. Promising targets particularly relate to the early detection of subjects at risk and monitoring of therapeutical efforts. Among other imaging modalities magnetic resonance imaging (MRI) is an emerging tool with strong potential and a favourable safety profile. This article summarizes the different approaches of imaging various facets of atherosclerotic disease by MRI. In particular, endothelial function, arterial stiffness, vessel remodeling, angiogenesis inside the vessel wall, vessel stenosis and plaque characterization are addressed. As such MRI is a very versatile diagnostic tool for vascular biology research with high diagnostic accuracy and reproducibility of its results. Moreover, MRI allows for comprehensive studies, applying several techniques within one exam.
- Copyright
- © 2008 Association for Research into Arterial Structure and Physiology. Published by Elsevier B.V. All rights reserved.
- Open Access
- This is an open access article distributed under the CC BY-NC license.
Introduction
In the past two decades, major advances in understanding the physiology and pathology of atherosclerotic disease have taken place. With increasing knowledge in vascular biology the former mechanistic view of the vessels and their pathology has changed to encompass a deeper understanding of functional aspects. It was found that the vessel wall with its complex physiology plays the major role in atherosclerotic disease. Diverse functional and morphologic manifestations have been found to occur with disease progression (see Fig. 1). Establishment of animal models of atherosclerosis has been a major step, that contributed to generation of knowledge about the impact of risk factors and effect of therapeutical measures, as well as the underlying genetic and biochemical causes. Endpoints are usually histological examination of the vessel wall after sacrificing the animal. Although imaging cannot equal a detailed histological examination, surrogate markers of the disease that can be imaged and quantified in vivo are of great interest, as they allow for serial studies and can potentially be translated to human applications.
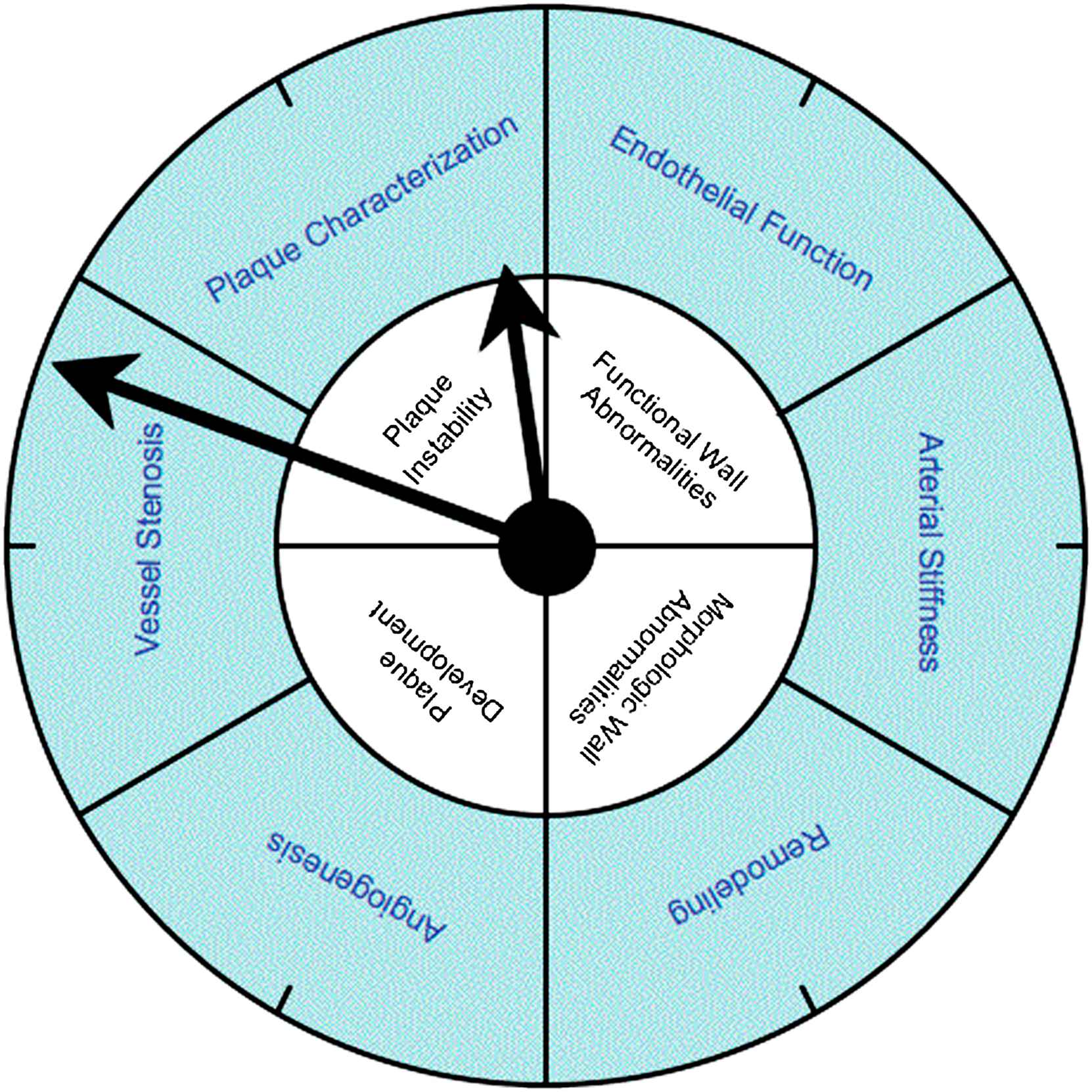
Atherosclerosis is illustrated in a clock to underline the goal of early detection. The labels in the inner circle reflect the different facets of atherosclerotic disease. Though they occur in a sequence of events, there is of course overlap and no strict progression. The labels in the outer circle represent the possible surrogate biomarkers of the disease as they can be targeted by magnetic resonance imaging.
The role of imaging
Atherosclerotic changes can be followed in vivo by angiography. This approach, however, displays intravascular lumen and does not provide information about the state and composition of the vessel wall.
Some vascular beds like the carotid vessels are also in the reach of high resolution ultrasound or can be reached using endoluminal and especially intravascular techniques. However, intravascular procedures are rather invasive and thus mostly confined to research.
Major advances have been achieved in the past years in computed tomography. Calcium in the vessel wall can be imaged and quantified reliably with electron beam or multislice spiral CT. There are also attempts to characterize non-calcified plaques by quantifying their attenuation of X-ray radiation. However, until now it is unclear whether this method is reliable and will ever be appropriate to be used as a clinical tool. In addition, CT still carries the drawback of relatively high dose exposure to radiation.
MRI has a favorable safety profile, is noninvasive and free of radiation (see Table 1). It can be applied to all organ systems and vessel beds. Aside from providing excellent anatomical detail the potential of tissue characterization deserves special attention. Contrast between and inside tissues can be obtained by the use of various sequences with a certain T1-, T2-, T2*- or proton-density-weighting. Additional contrast can be created by sophisticated tissue preparation with radiofrequency pulses and by the use of contrast agents.
Pros | Cons | |
---|---|---|
Angiography | High spatial and temporal resolution | Invasive |
Lots of experience | High radiation dose | |
Ultrasound | High spatial and temporal resolution | Operator dependent |
Lots of experience | Low potential for tissue characterization | |
Suitable for follow up (EXUS) | Invasive (IVUS) | |
Multislice computed tomography | High spatial resolution | Low temporal resolution |
Low operator dependence | High radiation dose | |
Non-invasive | ||
Magnetic resonance imaging | Non-invasive | Limited availability |
No radiation | ||
Low operator dependence | ||
High reproducibility | ||
Potential for tissue characterization | ||
Suitable for follow up |
EXUS: extravascular ultrasound; IVUS: intravascular ultrasound.
The pros and cons of the different imaging modalities in vascular biology
Major improvements in scanner technology as well as sequence development in the past years have improved both, spatial and temporal resolution. New applications emerged and MRI is increasingly used as a powerful diagnostic tool for imaging anatomy, structure and function of organs, including the blood vessels (see Fig. 1). This review highlights the major developments and applications of MRI as a diagnostic tool in vascular biology with a focus on those applications with potential relevance for clinical and research applications in humans.
Endothelial function assessment using MRI
The endothelial layer of the vessel wall plays a central role in vascular homeostasis. This includes the regulation of the vascular tone by endothelium-derived relaxing and contracting factors, as well as important effects on vascular inflammation, leukocyte adherence, mitogenesis, platelet activation, thrombosis and thrombolysis.1 Usually vasomotor function is used for assessing endothelial function as introduced for ultrasound by Celermajer et al. in 1992.2 The first MRI-based study on vasomotor function was published by Sorensen et al. in 2002.3 They used an identical setting as published by Celermajer et al., but employed high resolution MRI instead of ultrasound to assess flow mediated dilation (FMD) in the brachial artery (see Fig. 2). The use of a small surface coil and a segmented FLASH gradient echo sequence resulted in a pixel size of 0.27 × 0.27 mm and an acquisition time of 12 cardiac cycles. Measurements correlated well to intravascular ultrasound (IVUS) (r = 0.87, p < 0.01) and the interstudy reproducibility was significantly better for MRI. The most probable reasons for the improved reproducibility are that MRI is less operator dependent and truly cross sectional views of the vessel permit more accurate measurement. The lower interstudy variability allows for smaller sample sizes in controlled trials using FMD-related endpoints. MRI of FMD, however, is subjected to the same shortcomings as ultrasound: brachial artery dilation is dependent on shear stress and peripheral resistance, which are both influenced by interfering variables such as age, gender, hypertension and other risk factors.4 This problem was addressed by Silber et al. who implemented phase contrast magnetic resonance imaging to assess endothelial function.5 Using sequences with high temporal and spatial resolution they obtained a cross sectional flow map of a conduit artery. In each pixel, the peak flow velocity was determined. From the distribution of the peak flow velocities the radius of the vessel as well as the shear rate at the vessel wall can be calculated. FMD assessed with this method was well correlated to FMD assessed with ultrasound, but again interscan variability of CMR was smaller.6 The other advantage of this CMR approach is the possibility to simultaneously assess shear stress, which is the responsible variable for endothelial NO release and vasodilatation. This offers additional insights in interpreting FMD. Thus, Silber et al. were able to identify shear stress as a possible explanation for the greater FMD seen in smaller vessels.7 A limitation of this approach is, however, that it uses systolic peak flow, whereas the guidelines for FMD recommend diastolic assessment of vessel diameter.8
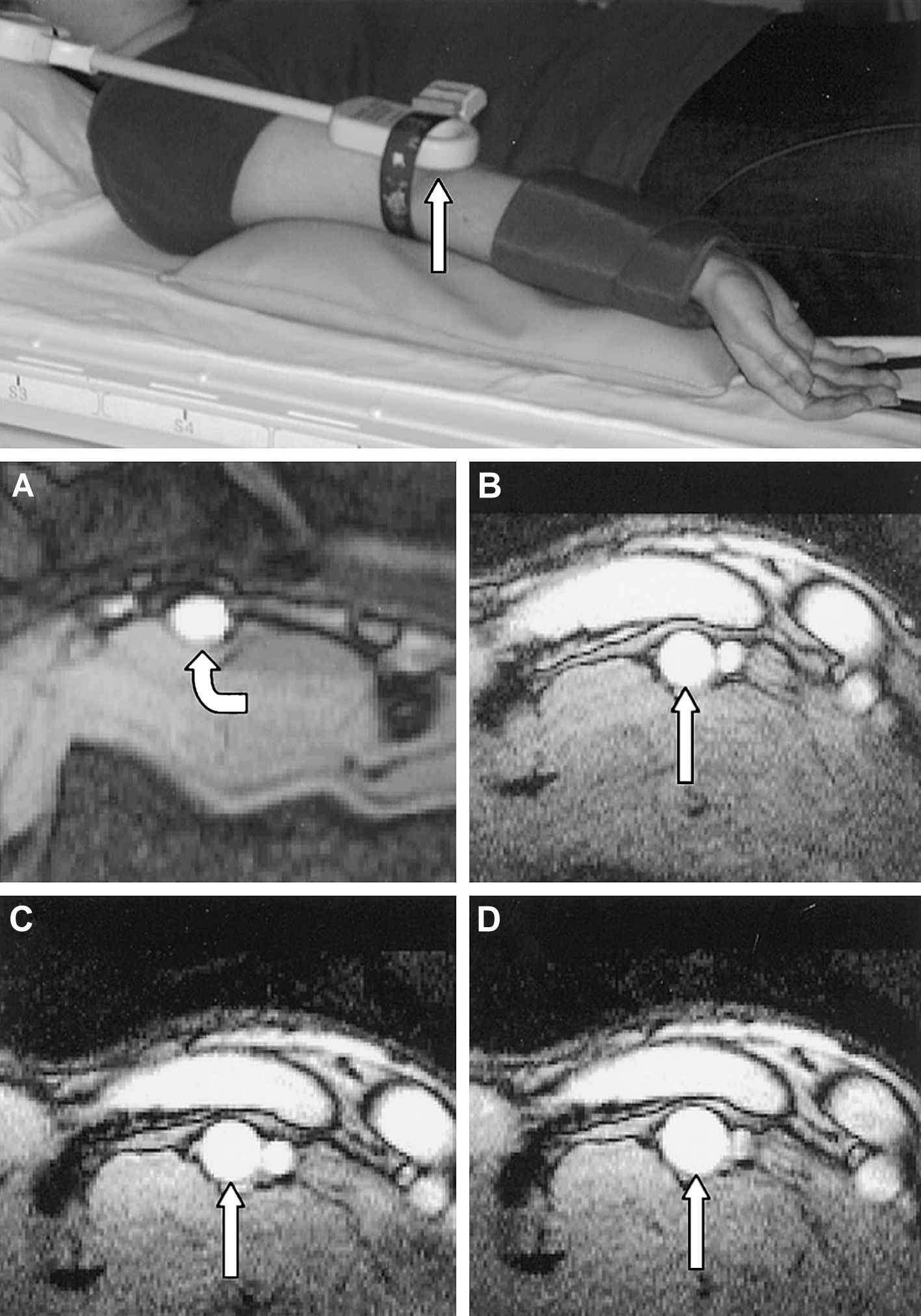
Use of a small surface coil in the set-up for assessing flow mediated dilation (FMD) by CMR (upper image). Baseline image of a brachial artery with an oval shape (A). (B–D): series of images in a healthy subject at baseline (B), during reactive hyperemia (C) and after sublingual dose of GTN (D) (figure reprinted with permission from Sorensen et al.3).
Schwitter et al. proposed a slightly different MR technique. An MR sequence for flow assessment with high temporal and spatial resolution allowed for direct assessment of flow and diameter changes in conducting arteries during reactive hyperemia.9 Flow changes during reactive hyperemia are considered to reflect endothelial function of resistance vessels. In a small sample, the group reported an association between MR assessed flow changes and cardiovascular risk.10
Another advantage of using MRI to assess endothelial function was demonstrated by Wiesmann et al. In a single, multimodal noninvasive MR study, they assessed peripheral endothelial function as introduced by Sorensen et al., as well as aortic and carotid distensibility and pulse wave velocity.11 All these variables are assessed simultaneously and are considered to be related to endothelial function.12
The utility of MRI to image other vessels than in the limb has been explored by Terashima et al.13 They used high resolution MRI to assess the vasodilatory capacity of the right coronary artery after sublingual nitroglycerin application. Though NO induced vasodilatation is not a standard test for endothelial function it is a first attempt to apply MRI to assess coronary vasodynamics in vivo.
Another possible approach to assess dynamic vasomotor effects could be blood-oxygen-level-dependent MRI (BOLD MRI). Signal intensity in BOLD MRI is dependent on the amount of deoxygenated hemoglobin in a tissue. Thereby the balance between oxygen supply and oxygen consumption can be imaged at tissue level. This effect is used in functional MRI of the brain.14 The principal ability of BOLD MRI to assess dynamic changes in oxygenation during endothelial function testing in the human forearm has been shown in a study by Utz et al.15 (see Fig. 3). It also has been applied in vivo for the diagnosis of myocardial ischemia.16
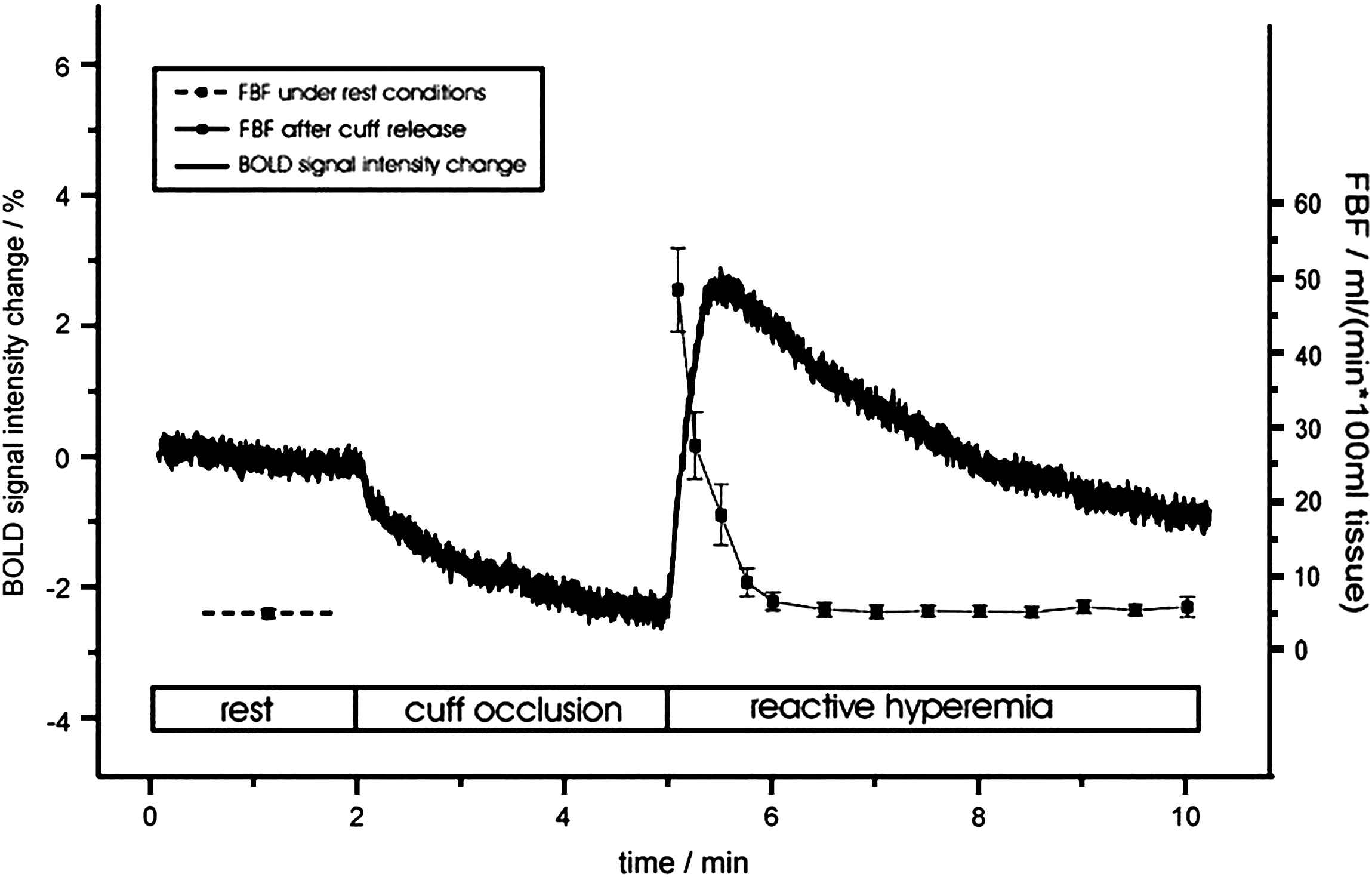
Signal changes in blood-oxygen-level-dependent (BOLD) MRI and blood flow (FBF) in a human forearm during reactive hyperemia after 3.5 min cuff occlusion. Note the different time scale of recovery to resting values for FBF and tissue oxygenation (figure reprinted with permission from Utz et al.15).
Arterial stiffness measured by MRI
Arterial stiffness is determined by morphological characteristics of the vessel wall, dependent upon the amount of elastin and collagen in the media. However, functional characteristics of the vessel wall such as endothelium dependent smooth muscle tone contribute as well to arterial stiffness. Both components may be altered during the course of atherosclerotic disease, and therefore arterial stiffness is considered a biomarker of atherosclerosis. However, its relation to other cardiovascular risk factors is not yet clear. Ultrasound-based methods as well as pulse wave analysis by tonometry and plethysmography to calculate the augmentation index have been applied to assess arterial stiffness. Another approach is to assess pulse wave velocity (PVW) as a function of arterial stiffness.
As early as 1989 Mohiaddin et al. presented an MR technique to assess aortic distensibility.17 As an advantage over ultrasound-based methods, all regions of the great vessels can be reached; moreover, it is less operator dependent than ultrasound. Other similar approaches were reported.18,19
Rogers et al. were the first to use MRI to assess pulse wave velocity (PWV).20 They could show regional differences in PWV in the aorta associated with age. A more sophisticated technique was presented by Yu et al.,21 who employed phase contrast images across the thoracic and abdominal aorta to calculate the pulse wave velocity within the aorta (see Fig. 4). This approach appears to be more objective because it does not rely on the subjective estimates of distances between carotid and femoral arteries as usually done in Doppler-ultrasound-based methods. In the same study, the aortic distensibility was also assessed from diameter changes of the aorta in relation to blood pressure changes during systole and diastole.
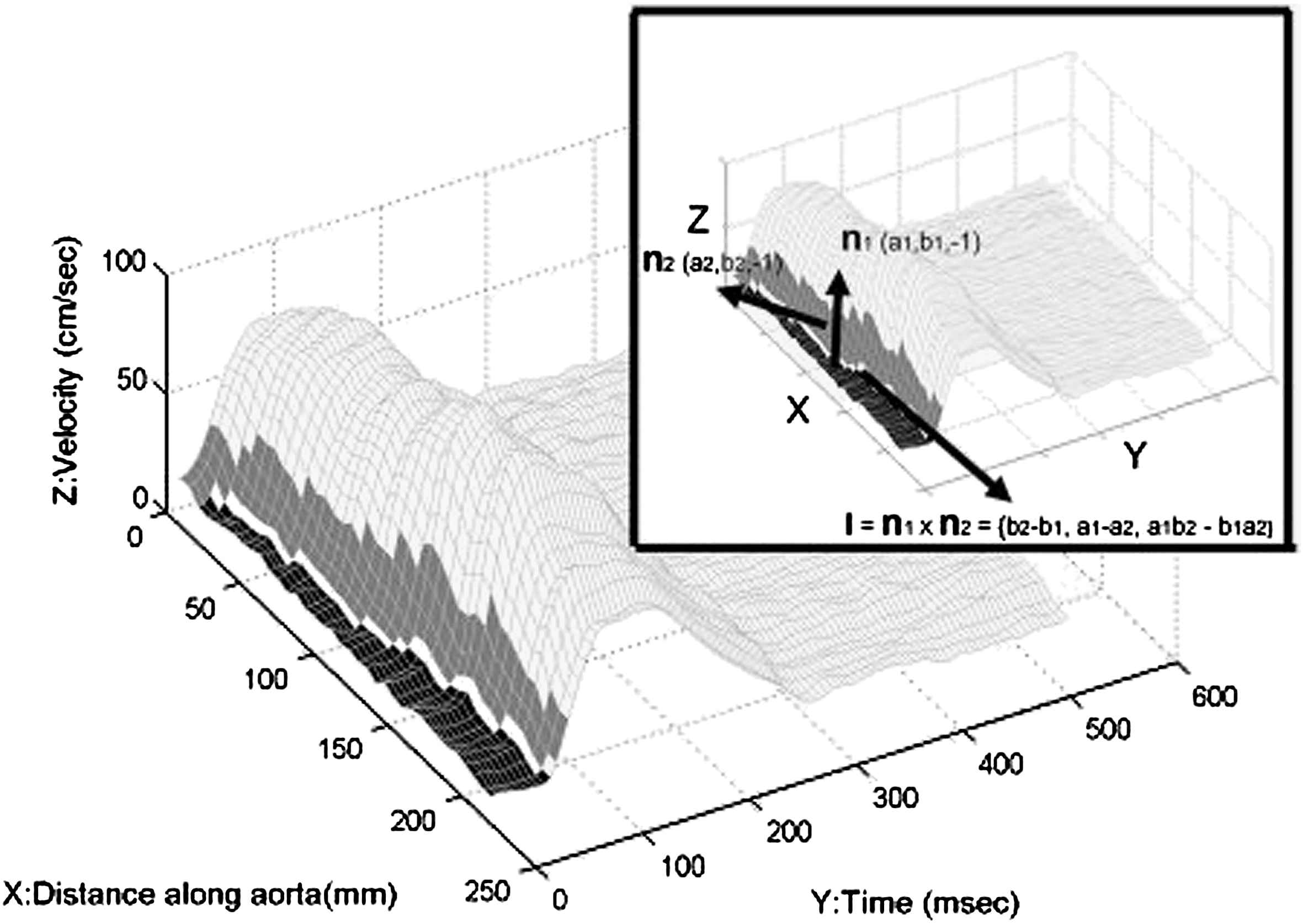
Pulse wave in consecutive sections of the aorta as determined by MRI. Pulse wave velocity is calculated as the slope of the vector I in the X/Y-plane (figure reprinted with permission from Yu et al.21).
Nevertheless, the role of arterial stiffness and pulse wave velocity as surrogate markers in atherosclerotic disease needs more clarification. Given the high accuracy and reproducibility of MRI this technique seems ideally suited to study arterial stiffness in arteriosclerotic disease in more detail.
Remodeling as assessed by MRI
It is well known that luminal angiography underestimates the true amount of atherosclerosis.22 One of the reasons for this is the extraluminal plaque extent and the increase in outer vessel diameter. This effect is referred to as vascular remodeling and was first described in human coronaries by Glagov et al.23 Positive remodeling is beneficial in terms of preventing flow limiting vessel stenosis. Surprisingly there is a huge variability of remodeling between individuals and between vascular beds in the same individual; the underlying causes and mechanisms are not clear.24 Probably inflammation and protease activation are involved.25 Methods for assessing remodeling have to be able to reliably quantify both, the intraluminal and extraluminal dimensions and structure of a vessel. Several animal studies have shown the ability of MR to follow vascular remodeling. Kaneko et al. performed balloon angioplasty in rabbits with induced atherosclerotic lesions in the aorta and iliac artery and were able to show with MRI positive remodeling thereafter.26 In another study on remodeling of the rabbit aorta after endothelial denudation, sequential MRI studies could show a significant increase in internal and external lumen after 6 months.27 Hegyi et al. induced atherosclerotic lesions in rabbits by balloon injury and high cholesterol diet. With high resolution MR (voxel size 0.25 × 0.25 × 2 mm) a significant increase of plaque volume was found after 12 weeks of a high cholesterol diet as well as the subsequent volumetric decrease during the following 12 weeks of regular feeding. Simultaneous increase of both plaque volume and intravascular lumen indicated positive vascular remodeling. Interestingly, this was detected as early as after 8 weeks of high cholesterol diet.28
Although different study protocols are needed, high resolution MRI can be applied to human subjects to assess internal and external vessel lumen as well as wall thickness29 (see Fig. 5). When these results are put into context of a normal vessel section in the same subject or of healthy control subjects positive remodeling can be demonstrated in humans as well.30
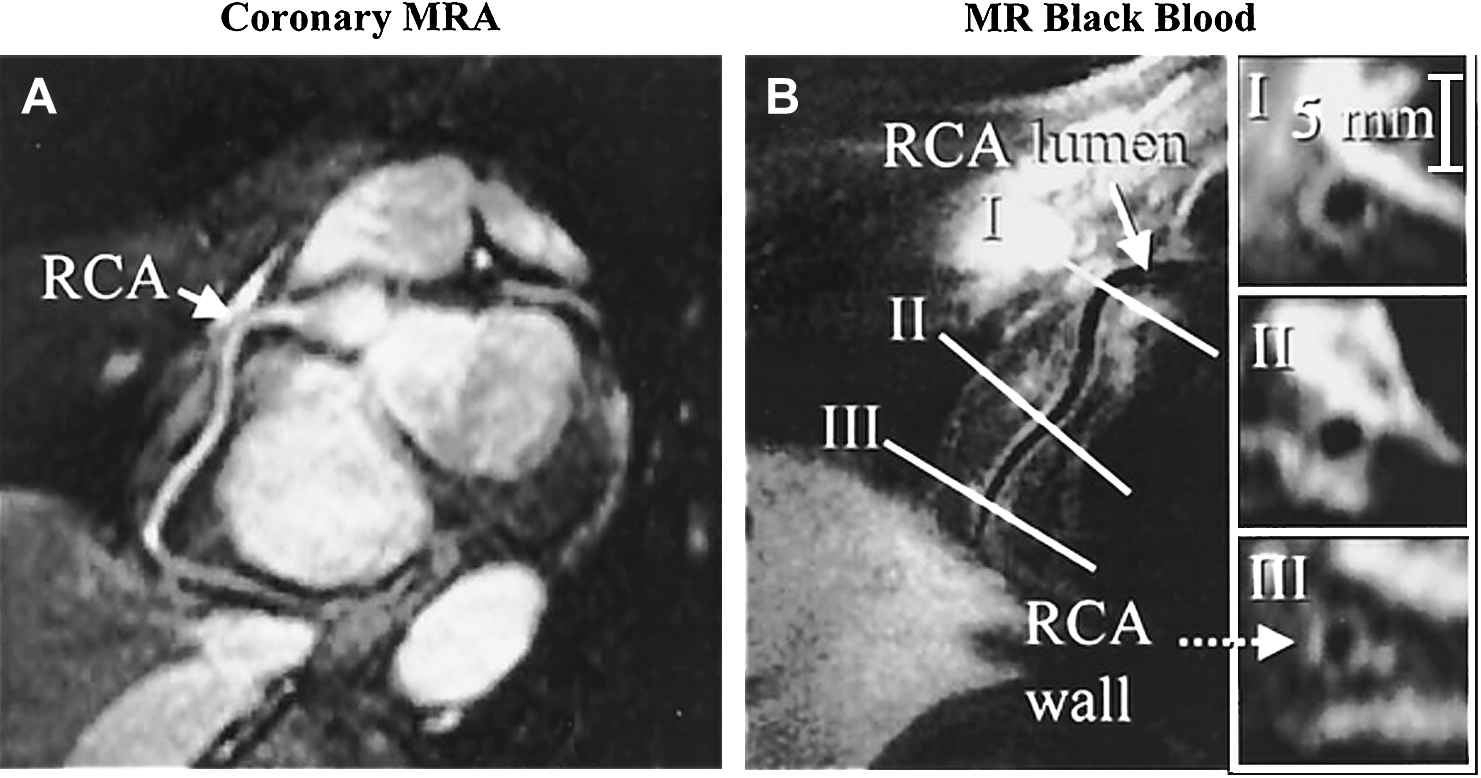
Reformatted 3D MR angiography of RCA (A) and arterial wall imaging in the double inversion dark blood technique (B). Note that though the wall is thickened in cross section I the lumen is not stenosed compared to the other cross sections due to positive remodeling (figure reprinted with permission from Kim et al.30).
Assessment of angiogenesis by MRI
Similar to cancer, proliferation processes play an important role in atherosclerosis. This applies to both, smooth muscle cells and the vasa vasorum, the vasculature of the vessels. In autopsy studies of patients with symptomatic atherosclerotic plaques an increased density of vasa vasorum has been found along with increased macrophage infiltration in these plaques.31 The same has been found in histological analysis of ruptured plaques in the human aorta.32 So angiogenesis is considered to play a relevant role in atherosclerotic disease.33
The permeability of the neovasculature in atherosclerotic plaques is increased compared to regular vessels. This leads to deposition of proteins like albumin and fibrin that promote inflammation and atherosclerosis. Accordingly, Gd-based contrast agents accumulate in plaques with prominent vasculature allowing for contrast-enhanced MRI studies. Carotid plaques with rich neovasculature have been reported to be identified with a sensitivity of 76% and a specificity of 79%.34
Dynamic contrast imaging can also be used to image the vasa vasorum. With fast T1 weighted gradient echo sequences a contrast bolus is tracked in the region of interest. The dynamics of T1 signal changes are dependent on multiple variables such as blood volume and flow, vessel density, capillary permeability, extravascular leakage space and of course the pharmacokinetic properties of the contrast agent. Kerwin et al. used this technique to detect and quantify the neovasculature in carotid plaques, observing a strong correlation to histologic findings.35 The same technique is also applied to image angiogenesis in cancer, especially during specific anti-angiogenesis therapy. However the technique is challenging because of confounding variables and the need of sophisticated algorithms for the analysis.36
Other approaches explore special properties of the intraplaque neovasculature such as an increased risk of rupture. Intraplaque hemorrhage as detected by high resolution MRI correlates with increased angiogenesis in the atherosclerotic wall.37 Intravascular hemorrhage has been found to correlate with more rapid plaque progression.38
Molecular imaging provides another fascinating and promising specific approach to highlight angiogenesis. Endothelial cells express integrin αvβ3 only during angiogenesis. This molecule can be targeted by MRI using labeled αvβ3-binding nanoparticles. Using this approach, Winter et al. have visualized vasa vasorum in plaques in rabbit aortas.39 This technique can be combined with therapeutic application of nanoparticles. If the targeted nanoparticles are additionally loaded with the anti-angiogenic fumagillin, the density of vaso vasorum in a rabbit model of atherosclerosis could be decreased40 (see Fig. 6). Similar approaches have been used for anti-angiogenic tumor therapy.41,42
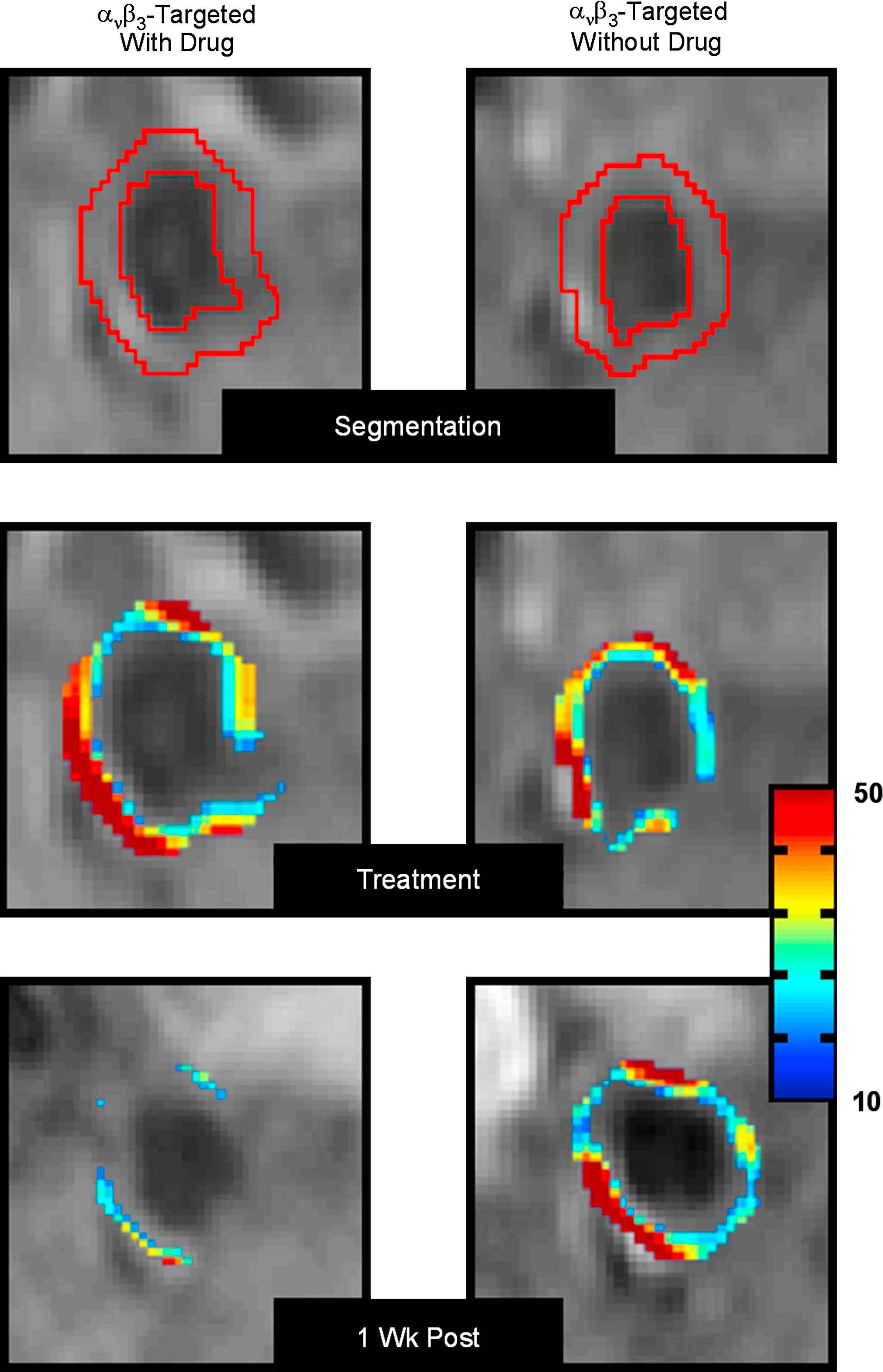
MRI of abdominal aorta of cholesterol fed rabbits (top panel). Coloured overlay in the middle panel represents signal enhancement 4 h after administration of αvβ3-targeted nanoparticles with (left) and without (right) fumagillin. The bottom panel shows the decrease in signal enhancement 1 week after fumagillin administration compared to control animals (figure reprinted with permission from Winter et al.40 (For interpretation of the references to colour in this figure legend, the reader is referred to the web version of this article.)
MRI of vessel stenosis
MR angiography has proven to be highly accurate in different vascular beds for the detection of relevant stenosis. Problems still exist in small vessels, peripheral stenosis and fibromuscular renal artery stenosis. The clinical value for the different vascular beds has extensively been reported elsewhere.43–47 As recently published whole body angiography can be performed in a single study with a 32 channel MR system with multicoil technology.48 Excellent results were reported with a sensitivity of 92% and a specificity of 96% in the symptomatic vascular territories compared to DSA.
Quantification of luminal coronary artery stenosis by MRI is challenging because of the small size and rapid motion of coronary arteries. Thus, a higher spatial and temporal resolution is needed which in turn increases imaging time far beyond that of a single breathhold. The use of respiratory-motion-triggered navigator techniques allows imaging during free breathing. For left main stenosis and 3-vessel disease a sensitivity of 100% and a specificity of 95% was reported in a multicentre trial.49 Further technical development improved image quality and shortened imaging time.50 The additional use of intravascular contrast agents does further improve image quality.51 However it has to be stated that at present the utility of MR angiography for quantifying coronary artery stenosis for the entire coronary artery tree is still far away from being diagnostic. Spatial and temporal resolution of MRI is not comparable so that considerable technical progress is needed to challenge the gold standard DSA.
Nevertheless, MRI has the advantages of being noninvasive and not using ionizing radiation. In addition, MRI allows for functional evaluation of stenosis such as myocardial or renal perfusion imaging. Considering the prognostic relevance of myocardial perfusion imaging and stress echocardiography52 and the surprisingly poor correlation of stenosis grade and perfusion defect,53 the functional rating of a stenosis is at least as relevant as its morphological appearance and quantification.
Plaque characterization by MRI
Atherosclerotic disease typically becomes symptomatic chronically with ischemic symptoms of a high grade stenosis or acutely if a thrombus suddenly forms and either embolizes or totally occludes a vessel. The latter is more drastic and often has fatal consequences for the downstream tissue. Usually an underlying, ruptured plaque is the cause for the thrombus formation.54 The preceding vulnerable plaque is histologically described as a plaque with a lipid core and a thin fibrous cap.55 Inflammatory processes and protease activation are thought to destabilize the fibrous cap and increase the risk of rupture. The detection of vulnerable plaques is therefore an important goal but still difficult to achieve. MRI is one of the most potent imaging modalities to detect vulnerable plaques.
Toussaint et al. were the first to demonstrate the feasibility of clinical MRI to distinguish between the components of a carotid plaque in vivo in humans. With a combination of T2-, T1- and proton density-weighted imaging they were able to identify the lipid core and the fibrous cap of plaques. Additionally, thrombotic plaque components, calcification as well as intraplaque hemorrhage could be identified.56 Several studies confirmed this ability of high resolution MRI. Even the differentiation between a thick fibrous cap, an intact thin fibrous cap and a ruptured fibrous cap has been shown to be possible, with high accuracy.57 With improving techniques the accuracy for the detection of the lipid core in carotid plaques can reach a sensitivity of 85% and specificity of 92%.58 Quantitative studies of plaque components such as calcification, loose matrix, hemorrhage and the thickness of the fibrous cap have shown a good correlation to histological findings59–61 (see Fig. 7). All these studies have been performed in vivo with clinical MR systems in carotid arteries of patients shortly before carotid thrombendarterectomy. Several techniques for plaque imaging in the aorta and coronary arteries have been published.62,63 Further improvement can be expected from better scanner and coil technology, rapid parallel imaging and novel techniques, such as diffusion weighted imaging. This includes an improvement of overall image quality64 and a sensitive detection of intravascular thrombosis.65 New contrast agents will provide further refinement in characterizing plaque components.34,66
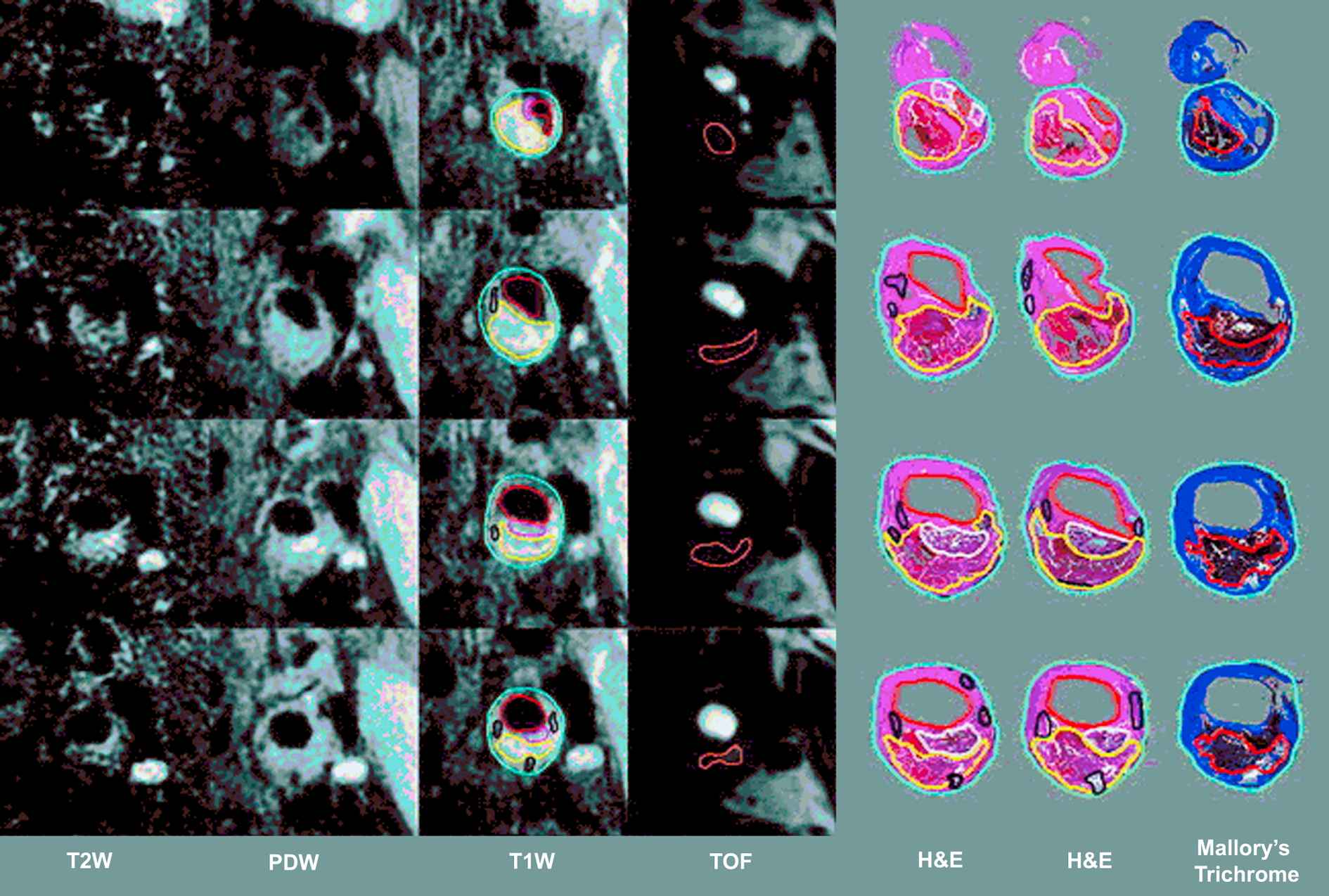
Comparison of MRI cross sections (2 mm thickness) in different techniques with histological sections in different stainings (H&E indicates hematoxylin–eosin). The contours are drawn for lumen (red), outer wall (cyan), lipid-rich/necrotic core (yellow), calcification (black), loose matrix (pink/white) and hemorrhage (orange) (figure reprinted with permission from Saam et al.59) (For interpretation of the references to colour in this figure legend, the reader is referred to the web version of this article.)
Two major clinical applications highlight the potential of these imaging techniques: First, the composition and the size of plaques can be monitored over time and therapeutic efficacy can be assessed in animal models67–69 and in humans.70–72 Compared to trials with clinical endpoints such as cardiovascular events the sample size of such studies can be significantly smaller.73 Though it seems reasonable to use plaque volume as a surrogate marker for atherosclerotic disease, it has to be considered that to date there are only a few studies on the correlation of plaque volume, or their composition, to cardiovascular events.74,75 Larger prospective trials are needed to confirm the feasibility of MRI to measure plaque volume as a clinical surrogate marker of atherosclerotic disease.
Second, patients at high risk with vulnerable plaques may in the future be identified for adjusting therapy. A thin fibrous cap and a large lipid core are considered indicators of a high risk plaque. Another potential marker of a vulnerable plaque is hemorrhage, leading to faster plaque progression.38 In particular, plaques with juxtaluminal hemorrhage are at risk, indicating plaque-erosion from the luminal side rather than rupture of a vessel inside the arterial wall.76
Whereas the described methods focus more on morphological properties of plaques, it is fascinating to target their biochemical and metabolic properties with molecular targeted MRI as an equivalent to immunohistochemical techniques. Using this approach macrophages in human carotid plaques could be targeted by ultrasmall superparamagnetic iron oxide (USPIO) which creates a signal decrease in macrophage infiltrated plaques with good correlation to histological control after thromboendarterectomy.77–79 Other approaches such as targeting macrophages with recombinant HDL nanoparticles, myeloperoxidase (MPO) activity with Gd-labeled substrates or fibrin by specific peptides or perflourocarbon nanoparticles seem to be efficient but are not yet approved in humans.80–83 The potential approaches of molecular imaging have recently been reviewed.84 Nevertheless it has to be stated that besides targeting macrophages with USPIO none of these approaches has yet been used and approved in human in vivo studies.
Summary
MRI is a versatile diagnostic tool for vascular biology studies. A large variety of structural and functional aspects of atherosclerotic disease can be imaged (see Fig. 1 and Table 2). Compared to other existing imaging modalities MRI often has superior diagnostic accuracy, and because of its safety, it can be used in clinical follow up studies. In addition, the ability to address different targets in multiple vascular beds in one MRI-study allows for a comprehensive, multimodal approach for studying experimental and clinical atherosclerotic disease.
Imaging target | Imaging modality |
---|---|
Endothelial function | Ultrasound (FMD) |
Angiography (ACH-test) | |
Magnetic resonance imaging | |
Arterial stiffness/pulse wave velocity | (Doppler) ultrasound |
Tonometry/plethysmography | |
Magnetic resonance imaging | |
Remodeling | Magnetic resonance imaging |
Multislice computed tomography | |
Angiography | |
Intravascular ultrasound | |
Angiogenesis | Magnetic resonance imaging |
Positron emission tomography | |
Ultrasound | |
Stenosis | Angiography |
Multislice computed tomography | |
Magnetic resonance imaging | |
Plaque characterization | Magnetic resonance imaging |
Intravascular ultrasound | |
Single photon emission computed tomography | |
Positron emission tomography |
The ranking reflects their current pertinence to the author’s perception (FMD: flow mediated dilation, ACH: acetylcholine).
The different imaging targets of atherosclerosis with their appropriate imaging modalities
MRI is less operator dependent than other techniques and has a higher reproducibility compared to other techniques. This allows smaller sample sizes in controlled trials. More than any other imaging technique, MRI may become the preferred noninvasive phenotyping tool for atherosclerotic disease.
Because MRI is a rather young technology, it still has a huge potential for further developments. Improved scanner technology, higher field strengths, new rapid imaging sequences and multichannel receiver coils will bring significant improvements in image quality in the near future. New contrast agents and new techniques for molecular imaging are other promising future developments.
References
Cite this article
TY - JOUR AU - Matthias Voehringer AU - Udo Sechtem AU - Matthias G. Friedrich PY - 2008 DA - 2008/02/29 TI - Magnetic resonance imaging in vascular biology☆ JO - Artery Research SP - 9 EP - 20 VL - 2 IS - 1 SN - 1876-4401 UR - https://doi.org/10.1016/j.artres.2008.01.002 DO - 10.1016/j.artres.2008.01.002 ID - Voehringer2008 ER -