Sodium excretion as a modulator of genetic influence on arterial stiffness and other cardiovascular phenotypes
First presented at Artery 6, Athens, Greece, 2223 September 2006
- DOI
- 10.1016/j.artres.2007.03.002How to use a DOI?
- Keywords
- Genetics; Arterial stiffness; Left ventricular mass; Heart rate variability; Sodium
- Abstract
Hypertension is a chronic age-related disorder, affecting nearly 20% of all adult Europeans. This disease entails debilitating cardiovascular complications and is the leading cause for drug prescriptions in Europeans older than 50 years. Intensive research over the past two decades has so far failed to identify common genetic polymorphisms with a major impact on blood pressure or associated cardiovascular phenotypes, suggesting that multiple genes each with a minor impact, along with gene–gene and gene–environment interactions, play a role. The European Project on Genes in Hypertension (EPOGH) is a large-scale, family-based study in which participants from seven different populations were phenotyped and genotyped according to standardized procedures. The EPOGH demonstrated that phenotype–genotype relations strongly depend on host factors such as gender and lifestyle, in particular salt intake as reflected by the 24-h urinary excretion of sodium. Individuals with the same genetic predisposition had different vascular stiffness, left ventricular mass or heart rate variability, depending on whether they ate a high-sodium or a low-sodium diet. The EPOGH therefore highlights the concept that phenotype–genotype relations can only be studied within a defined ecogenetic context.
- Copyright
- © 2007 Published by Elsevier B.V. on behalf of Association for Research into Arterial Structure and Physiology.
- Open Access
- This is an open access article distributed under the CC BY-NC license.
Introduction
Cardiovascular disorders are the direct cause of death for over 60% of Europeans. Hypertension, a chronic age-related disorder, affects nearly 20% of all adults, entails debilitating cardiovascular complications and is causally involved in nearly 70% of all strokes.1 From the viewpoint of health economics, both in Europe and the United States, hypertension is the leading cause for drug prescriptions in patients older than 50 years.2
Hypertension arises through the complex interaction between genetic, environmental and lifestyle factors.3 Another approach to identify genetic causes of disease is to search for association with specific candidate genes. More than 150 candidate genes have been studied in relation to hypertension and other cardiovascular phenotypes. Often, strong associations are reported that are not confirmed in subsequent studies. However, a negative finding or a minor genetic effect in a general population may become a major gene effect in a subgroup of people with the appropriate genetic and environmental background. This can occur because of the network of feedback mechanisms regulating complex traits. Gene–environment and gene–gene interactions (biological) are currently a topic of great interest in the field of genetic epidemiology. Better accounting for these interactions probably holds promise for advancing our understanding of the pathophysiology of blood pressure and related cardiovascular phenotypes.
The European Project on Genes in Hypertension (EPOGH) is a European family-based epidemiological survey with the following objectives: to search for interactions between genes and the environment in the pathogenesis of hypertension and related intermediate phenotypes; and to produce a representative population-based database of complex cardiovascular phenotypes in relation to multiple genotypes and environmental factors. This review, based on data from the EPOGH, will focus on the empirical observations of the interactions between different cardiovascular phenotypes, the genes encoding various components of the renin–angiotensin–aldosterone system (RAAS) and the adducin cytoskeleton, and sodium excretion as an index of salt intake. The hypothesis of effect modification of the aforementioned genes by sodium intake was made a priori for the following reasons: RAAS and adducin play a key role in sodium homeostasis4,5; and, via various mechanisms, high sodium might affect blood pressure and sympathetic tone, promote cardiac growth and change wall properties of the large arteries.6–8
Arterial wave reflection
Angiotensin II and aldosterone generated by the angiotensin-converting enzyme (ACE) and aldosterone synthase, respectively, not only regulate sodium and water homeostasis, but also influence vascular remodeling in response to high blood pressure. In 622 EPOGH subjects from three European populations, we therefore investigated whether the ACE D/I and CYP11B2 C −344T polymorphisms influence arterial wave reflections, a measure of vascular stiffness.9 The peripheral and central augmentation indexes were significantly higher in CYP11B2 −344C allele carriers than in −344T homozygotes in population-and family-based analyses. However, this effect of the CYP11B2 polymorphism only occurred in subjects with a higher than median urinary sodium excretion (210 mmol/day). The association between systolic augmentation and the ACE D/I polymorphism did not reach statistical significance.9 Previous studies demonstrated that, in humans, a high salt intake is associated with increased arterial stiffness and vascular hypertrophy.8 Furthermore, stroke-prone spontaneously hypertensive rats fed 0.9% NaCl in drinking water, compared with a control group given tap water, had increased expression of mRNA for CYP11B2 in the arterial wall, but lower levels of circulating aldosterone.10 Thus, an excessive salt intake might contribute to increased arterial stiffness by inappropriately sustaining the expression of the CYP11B2 gene in the arterial wall, especially in −344C allele carriers.
In a population sample of 1069 untreated subjects we investigated the relation between arterial characteristics and renal sodium handling as assessed by the clearance of endogenous lithium. We ultrasonographically measured diameter, cross-sectional compliance (CC) and distensibility (DC) of the carotid, brachial, and femoral arteries. While accounting for covariates and standardizing for the sodium excretion rate in both sexes, CC and DC of the femoral artery increased with higher fractional distal sodium reabsorption. Differences associated with a 1-SD change in fractional distal reabsorption of sodium were 51.7 mm2/kPa × 10−3 (95% CI: 23.9–79.5; P = 0.0002) and 0.56 × 10−3/kPa (95% CI: 0.17–0.94; P = 0.004) for femoral CC and DC, respectively. In women as well as in men, a 1-SD increment in fractional proximal sodium reabsorption was associated with decreases in femoral and brachial diameter, amounting to 111.6 μm (95% CI: 38.2–185.1; P = 0.003) and 52.5 μm (95% CI: 10.0–94.9; P = 0.016), respectively. There was no consistent association between the properties of the elastic carotid artery and renal sodium handling. These findings demonstrate that there might be an influence of renal sodium handling on arterial properties or vice versa or that common mechanisms might influence both arterial and renal function.11
Left ventricular structure
Angiotensin II and aldosterone, also influence cardiac remodeling.12 We therefore investigated genetic polymorphisms of ACE, the angiotensin II type 1 (AGTR1) and type 2 (AGTR2) receptors and aldosterone synthase (CYP11B2) in relation to the left ventricular mass index (LVMI), analyzed as a continuous trait. Overall, these analyses included about 820 subjects from 220 nuclear families from Cracow (Poland), Novosibirsk (Russia) and Mirano (Italy).13,14
We first tested heterogeneity across the three populations. We found significant differences between the two Slavic centers and Mirano in LVMI (94.9 versus 80.3 g/m2), sodium excretion (229 versus 186 mmol/day), and the prevalence of the ACE D allele (52.1 versus 58.5%) and the AGTR2 A allele (45.8 versus 55.1%). There was significant heterogeneity between Slavic and Italian subjects in the phenotype–genotype relations with the ACE gene, but not with the aldosterone synthase or AGTR genes.
The D/I polymorphism, an insertion or deletion of 287 base pairs at intron 16 of the ACE gene on chromosome 17, is the most frequently investigated polymorphism in relation to left ventricular mass.15 Our results demonstrated that in the two Slavic centers ACE II homozygosity was significantly associated with higher LVMI in population- and family-based analyses. By contrast, in Mirano, LVMI was slightly higher in DD homozygotes (P = 0.05), but only in the population-based approach.13 In line with published data,16 we demonstrated that ACE II homozygotes had lower plasma ACE activity than D-allele carriers. We speculated that the margin of adaptation of the RAAS in response to chronic high salt intake, as observed in Slavic populations, might be smaller in II homozygotes than in D-allele carriers. In line with this hypothesis, some investigators found that patients homozygous for the I allele experienced a significantly greater rise in blood pressure in response to a high salt intake compared with DD homozygotes.17,18 Impaired suppression of the RAAS might stimulate left ventricular hypertrophy.19 Thus, the relation between the LVMI and the ACE D/I polymorphism differs across populations, possibly because of intermediate regulatory mechanisms responsive to varying levels of salt intake.
In the EPOGH cohort, within and across populations, we did not find any relation between the LVMI and the urinary excretion of aldosterone or the CYP11B2 −344C/T polymorphism.20 The interaction between the CYP11B2 −344C/T polymorphism and sodium excretion in relation to the LVMI did not attain statistical significance (P = 0.08). However, we measured only one single nucleotide polymorphism in the promoter area of the gene and we can therefore not exclude that full assessment of CYP11B2 haplotypes would have yielded stronger relations with the cardiac phenotypes.
As angiotensin II acting through the AT1 receptor stimulates sodium reabsorption and cell growth, the hypothesis that AGTR1 gene variants might be associated with left ventricular mass is plausible. Many polymorphisms of AGTR1 have been identified, but the A1166C polymorphism has been most extensively studied in relation to blood pressure, arterial stiffness and left ventricular mass. Although this variant is located in the 30-untranslated region of the gene, it might be in linkage disequilibrium with a hitherto undiscovered functional variant. In our study, the AGTR1 A1166C polymorphism was unrelated to the LVMI, even if we accounted for sodium excretion.14 In keeping with the present observations, most,21,22 but not all,23 clinical researchers were unable to demonstrate an association between left ventricular mass and the AGTR1 A1166C polymorphism. This might be due to insufficient sample size in echocardiographic studies or the low prevalence of the CC genotype.
The AT2 receptor probably counterbalances the vasoconstrictor and anti-natriuretic effects produced by angiotensin II via the AT1 receptor.24 The AGTR2 gene maps to the X chromosome.24 Regulatory elements of the transcription of AGTR2 are located in the promoter area and the first intron.25 The AGTR2 G1675A polymorphism, which is located in intron 1, is likely to be functional in that the G allele is probably associated with increased AGTR2 transcription and therefore with increased expression of the AT2 receptor. We observed that in men the effect of the AGTR2 G1675A polymorphism on the left ventricular mass differed according to sodium excretion.14 In women, this gene–environment interaction did not reach statistical significance. Continuous analyses demonstrated that in male G-allele carriers the LVMI and left ventricular internal diameter, but not the wall thickness, increased with higher sodium excretion. Further analyses involving only untreated men and dichotomized according to the median sodium excretion showed that, when sodium excretion was less than 240 mmol/day (median), the LVMI was lower in G-allele than A-allele carriers.14
Only few studies have addressed the possible association of left ventricular mass with the AGTR2 G1675A polymorphism in humans.26,27 In accordance with our findings, the G-allele frequency in these studies varied from 43 to 51%. In 120 men with normal or mildly elevated blood pressure and a mean age of 26 years, Schmieder et al.26 found that hypertensive but not normotensive G-allele carriers had a lower left ventricular mass than A-allele carriers, due to a reduced wall thickness. Herrmann et al.27 observed a higher prevalence of the G allele in 55-year-old to 74-year-old Scottish men (n = 336) without electrocardiographic left ventricular hypertrophy enrolled in the GLAOLD study compared with those with hypertrophy. However, these researchers could not confirm their findings using electrocardiographic and echocardiographic phenotyping in 629 men, 25–74 years old, investigated in the framework of the GLAECO study,27 even if the subjects older than 55 years were studied separately.
None of the previous studies in humans accounted for sodium intake. The hypothesis that the AT2-mediated effects of angiotensin II on the LVMI are modulated by sodium intake is plausible, although the exact mechanism remains to be elucidated. Indeed, in animal experiments, dietary sodium depletion enhanced the expression of AT2 receptors.28 The latter may play a counter-regulatory role that opposes the sodium-retaining effect of angiotensin II mediated via the AT1 receptor. Moreover, in sodium-depleted rodents, stimulation of the AT2 receptor produced natriuresis,29 whereas the opposite might occur in sodium-replete animals.30
The left ventricular mass is calculated from the left ventricular internal diameter and the wall thickness. The left ventricular diameter, to some degree, reflects the circulating fluid volume, whereas the wall thickness might be more indicative of processes confined to the myocardium itself. We found that the interaction between the G1675A polymorphism and sodium excretion in relation to the LVMI was mediated via the left ventricular internal diameter. This suggests that renal AT2 receptors, via their influence on sodium balance and the circulating plasma volume, might also contribute to the explanation of our findings. On the other hand, we also confirmed an independent effect of the ACE D/I genotype on the LVMI. As we noticed in a previous publication,13 this effect was mediated via wall thickness and it was also dependent on sodium intake. Thus, overall, our findings support the hypothesis that the AGTR2 G1675A and the ACE D/I polymorphisms independently influence the left ventricular mass, and that salt intake modulates this genetic effect. However, because of the exploratory nature of our analyses, which is inherent to all observational studies, our findings need replication in future human and experimental research.
Heart rate variability
Sympathetic tone increases with stimulation of the RAAS and is under the influence of salt intake.31 We therefore investigated whether polymorphisms in the genes encoding aldosterone synthase (CYP11B2 C −344T) and the type-1 angiotensin II receptor (AGTR1 A1166C) affect the autonomic modulation of heart rate variability at varying levels of salt intake.32 We measured the low-frequency (LF) and high-frequency (HF) components of heart rate variability and their ratio (LF:HF) in the supine and standing positions in 1797 participants (401 families), randomly recruited from six European populations, whose average urinary sodium excretion ranged from 163 to 245 mmol/day. When we did not account for sodium excretion, neither the population-based nor the family-based analyses revealed any significant association between heart rate variability and the genetic polymorphisms. However, in subjects with sodium excretion below 190 mmol/day, the supine heart rate, LF component and LF:HF ratio increased and the HF component decreased with the number of CYP11B2 −344T alleles (Fig. 1). The orthostatic changes in the LF, HF and LF:HF components were blunted in carriers of the AGTR1 1166C allele. In subjects with sodium excretion above 190 mmol/day, these associations with the CYP11B2 and AGTR1 polymorphisms were non-significant or in the opposite direction, respectively (Fig. 1).
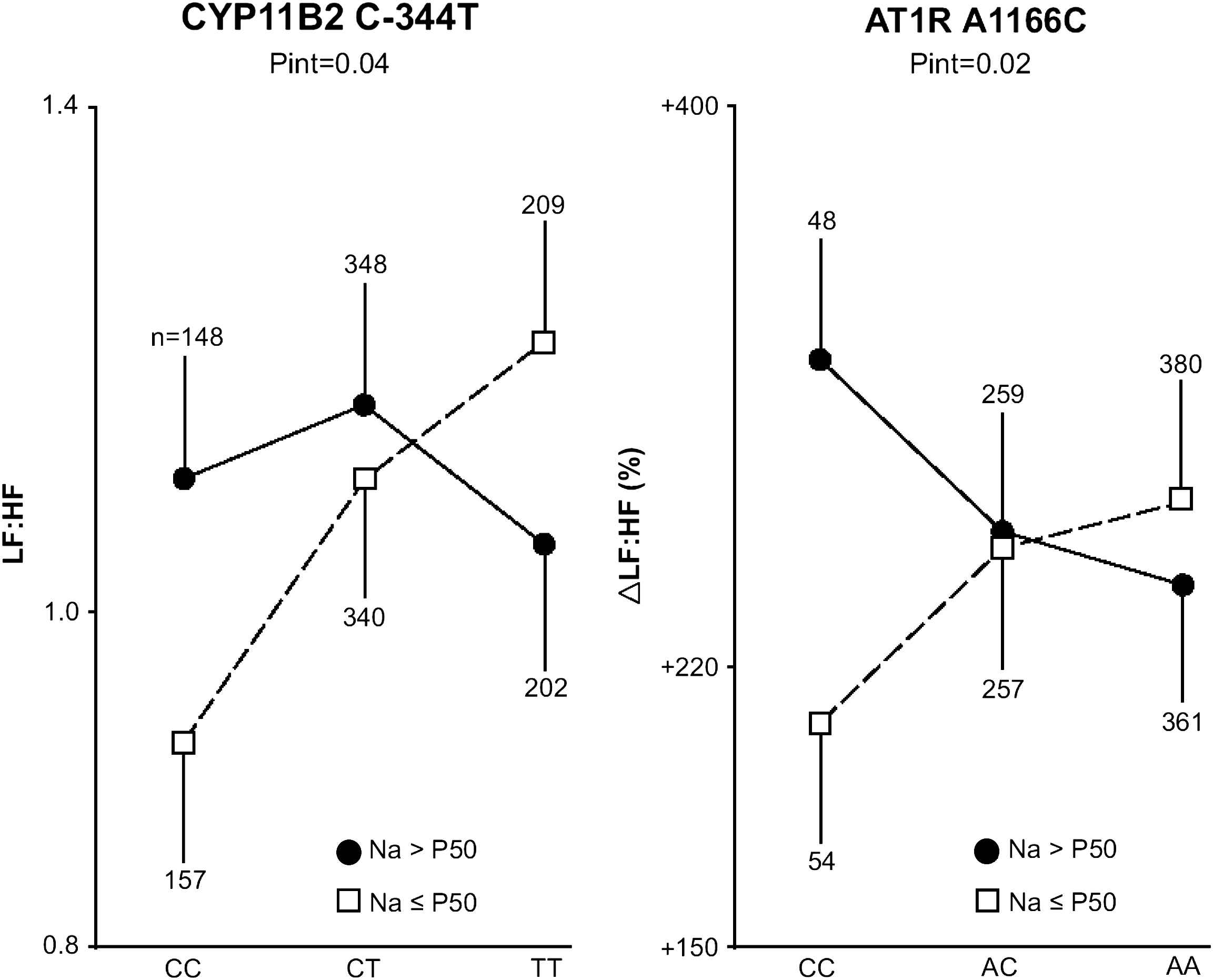
Low-to-high frequency ratio in the supine position (LF:HF) and orthostatic change in this ratio expressed as a percentage of its value in the supine position (ΔLF:HF) in relation to the CYP11B2 and AT1R polymorphisms, respectively. Associations were plotted for 2 groups, based on country- and sex-specific medians of sodium excretion. The probability of the interaction (Pint) between genotype and sodium excretion analyzed as a continuous variable was derived by GEE and accounts for non-independence within families and covariates.
Our findings,32 in keeping with other reports in the literature,33,34 support the hypothesis that genetic polymorphisms and lifestyle factors leading to expansion of the circulating plasma volume might affect the autonomic nervous regulation of the cardiovascular system. An excessive salt intake might be associated with an expanded circulating plasma volume,35 which in turn might mask the genetic influence of the CYP11B2 C −344T polymorphism on heart rate variability. Salt intake modulates the expression of AT1 receptors.36 Depending on salt intake, the AGTR1 1166CC genotype may or may not be associated with a tendency for volume expansion, which might explain the differential autonomic modulation of heart rate variability in CC homozygotes. Thus, the CYP11B2 C −344T and AGTR1 A1166C polymorphisms affect the autonomic modulation of heart rate variability in the supine and standing positions, respectively, but these genetic effects depend on sodium intake.
Conclusions
The EPOGH demonstrated that individuals with the same genetic predisposition had different vascular stiffness,9,11 left ventricular mass13,14 or heart rate variability32 depending on dietary sodium intake. As exemplified by the present results, environmental factors modify the actions of genes. Thus, population studies that take into account gene–environment and gene–gene interactions will increasingly be used to study complex cardiovascular phenotypes.
Acknowledgements
The European Union (IC15-CT98-0329-EPOGH, QLGI-CT-2000-01137-EURNETGEN and LSHM-CT-2006-037093) provided support for the recruitment and phenotyping of families. Research included in the present article was funded by grants (G.0291.98, G.0424.03, G.0453.05) from the Fonds voor Wetenschappelijk Onderzoek Vlaanderen (FWO, Brussels, Belgium), by special research grants (Onderzoekstoelage OT/99/28, OT/00/25, OT/05/49) from the Katholieke Universiteit Leuven (Leuven, Belgium), and by the International Scientific Collaboration between Poland and Flanders contract numbers BIL 00/18 and BIL 05/22).
Appendix EPOGH centers
Belgium (Leuven) – E. Balkestein, R. Bollen, H. Celis, E. Den Hond, A. Hermans, L. De Pauw, P. Drent, D. Emelianov, R. Fagard, L. Gijsbers, T. Nawrot, L. Thijs, Y. Toremans, J. A. Staessen, S. Van Hulle, J. G. Wang, R. Wolfs.
Bulgaria (Sofia) – C. Nachev, A. Postadjian, E. Prokopova, E. Shipkovenska, K. Vitljanova.
Czech Republic (Pilsen) – J. Filipovský, J. Kucerova, V. Svobodova, M. Tichá.
Czech Republic (Prague) – O. Beran, L. Golán, T. Grus, J. Peleška, Z. Marecková.
Italy (Padova) – E. Casiglia, A. Pizzioli, V. Tikhonoff.
Poland (Cracow) – A. Adamkiewicz-Piejko, M. Cwynar, J. Gąsowski, T. Grodzicki, K. Kawecka-Jaszcz, W. Lubaszewski, A. Olszanecka, K. Stolarz, B. Wizner, W. Wojciechowska, J. Życzkowska.
Romania (Bucharest) – S. Babeanu, D. Jianu, C. Sandu, D. State, M. Udrea.
Russian Federation (Novosibirsk) – T. Kuznetsova, S. Malyutina, Y. Nikitin, E. Pello, M. Ryabikov, M. Voevoda.
Co-ordination
Project co-ordinator – J. A. Staessen.
Scientific co-ordinator – K. Kawecka-Jaszcz.
Steering committee – S. Babeanu, E. Casiglia, J. Filipovský, K. Kawecka-Jaszcz, C. Nachev, Y. Nikitin, J. Peleška, J. A. Staessen.
Data management – T. Kuznetsova, J. A. Staessen, K. Stolarz, L. Thijs, V. Tikhonoff, J. G. Wang.
Molecular biology – G. Bianchi (Milan), E. Brand (Berlin, Münster), S. M. Brand-Herrmann (Münster), H. A. Struijker-Boudier (Maastricht).
Echocardiography – E. Casiglia (Padova), K. Kawecka-Jaszcz (Cracow), Y. Nikitin (Novosibirsk).
Vascular phenotypes – K. Kawecka-Jaszcz (Cracow), J. Filipovský (Pilsen), J. A. Staessen (Leuven), H. A. Struijker-Boudier (Maastricht), L. Van Bortel (Ghent).
EPOGH–EurNetGen liaison – A. Dominiczak (Glasgow), J. A. Staessen (Leuven).
References
Cite this article
TY - JOUR AU - Katarzyna Stolarz AU - Tatiana Kuznetsova AU - Wiktoria Wojciechowska AU - Jitka Seidlerova AU - Edoardo Casiglia AU - Jan Filipovský AU - Jan Peleška AU - Yuri Nikitin AU - Jan A. Staessen AU - Kalina Kawecka-Jaszcz AU - on behalf of the European Project On Genes in Hypertension (EPOGH) Investigators PY - 2007 DA - 2007/06/06 TI - Sodium excretion as a modulator of genetic influence on arterial stiffness and other cardiovascular phenotypes JO - Artery Research SP - 20 EP - 25 VL - 1 IS - 1 SN - 1876-4401 UR - https://doi.org/10.1016/j.artres.2007.03.002 DO - 10.1016/j.artres.2007.03.002 ID - Stolarz2007 ER -