Applanation tonometry for evaluation of the haemodynamic response to the active orthostatic test
- DOI
- 10.1016/j.artres.2017.07.001How to use a DOI?
- Keywords
- Arterial stiffness; Pulse wave analysis; Active orthostatic test; Augmentation index; Heart rate variability
- Abstract
Background: Our study was aimed at the assessment of the age-specific hemodynamic response to the active orthostatic test (OT).
Methods: Two groups (60-Y/O and 20-Y/O) were subjected to OT (three 5-minute periods: baseline supine, upright standing, recovery supine). Continuous electrocardiogram was recorded and heart rate variability (HRV) analysed. At the end of each period pulse wave analysis was performed (Sphygmocor, AtCor Medical).
Results: We observed significantly higher radial and central systolic and pulse pressures in the adults at baseline. We found out reduced efficiency of the hemodynamic compensatory response to orthostasis in the 60-Y/O group: mild heart rate increase (75 ± 2 vs. 88 ± 3 beats per minute); moderate aortic systolic pressure reduction (124 ± 6 to 119 ± 5 vs. 99 ± 2 to 101 ± 3 mm Hg); considerable aortic pulse pressure decrease (47 ± 4 to 38 ± 3 vs. 27 ± 2 to 22 ± 2 mm Hg); absence of diastolic pressure elevation. The adults showed significantly higher baseline augmentation indices (AIx); the young responded to the upright position with noticeable reduction of AIx most probably resulting from the pronounced tachycardia and the consequent delayed timing of pulse wave return. HRV data showed milder sympathetic activation in the 60-Y/O individuals in response to OT as compared to the 20-Y/O.
Conclusions: Pulse wave analysis provided new knowledge concerning the age-related hemodynamic response pattern to active orthostasis. We believe the underlying mechanism was the ‘physiological’ arterial stiffening with aging and the ensuing reduced arterial baroreflex sensitivity. HRV data for lesser sympathetic activation in response to the orthostatic challenge in the 60-Y/O group supported this assumption.
- Copyright
- © 2017 Association for Research into Arterial Structure and Physiology. Published by Elsevier B.V. All rights reserved.
- Open Access
- This is an open access article distributed under the CC BY-NC license.
Introduction
Cardiovascular diseases are still the leading cause of morbidity and mortality worldwide.1 To reduce this burden, it is of great importance to reveal the primary risk factors and to focus prevention on the individuals at higher cardiovascular risk. It was clearly shown that the predictive force of individual or combined cardiovascular risk factors was not satisfactorily high.2 Arterial stiffness was evidenced to reflect the damaging effects of various noxae on the arterial wall: elevated arterial pressure, overweight or obesity, hyperglycemia, dyslipidemia, etc. All these factors contribute to the ‘physiological’ ageing of the vessels, especially of the elastic arteries, and thus accelerate their stiffening. Arterial stiffness is assumed to be a composite measure of the inherited (non-modifiable) risk factors as well as the long-lasting impairing modifiable influences. Therefore, arterial stiffness was recognised as the most potent tool for screening the individuals at higher cardiovascular risk.3
Two are the most popular methods for the non-invasive evaluation of arterial stiffness. Carotid-femoral pulse wave velocity (PWV) has been evidenced to be the “gold standard” for assessment of central arterial stiffness.4 Relevant information might be obtained by the analysis of the central pulse wave (PWA) as well.5,6
The modern non-invasive method applanation tonometry provides reproducible data for PWA of the central pressure waveform. This method presents the aortic pulse wave based on the peripheral arterial pressure record using a validated transfer function.7 The central pulse waveform results from the interaction between the forward (incident) wave and the reflected pulse wave from the periphery. The amplitude of the forward wave depends primarily on the myocardial contractility and the aortic compliance. The amplitude of the reflected pulse wave depends on the compliance of the arterial circulation, mainly on the compliance of the more distal arteries, on the peripheral arterial resistance and on the calibre and the impedance mismatch between the central and peripheral arteries. In fact, it is not one single wave that is reflected; numerous ‘wavelets’ start at different reflection sites and are propagated back towards the aortic valve.8 The cumulative reflected wave is superimposed on the incident wave. The timing of the reflected wave return depends on the arterial viscoelastic properties: stiffened arteries conduct pulse wave with higher velocity.9 The exact timing depends on the heart rate and the height of the individual as well.10–12 Therefore, besides early return of the reflected pulse wave in case of stiffened arterial wall, bradycardia and shorter stature might be expected to cause relatively earlier timing of the pulse wave return in the cardiac cycle. All these intricate correlations finally determine the specific central pulse waveform pattern. In the young individuals the return of the reflected wave happens usually during diastole; consequently, the systolic peak P1 is higher than P2 and coincides with the incident wave. This type of aortic pulse tracing was postulated to be of type C. In the older people the reflected wave returns early in systole resulting in a higher peak P2 than P1 and generates the type A pulse waveform.13
Numerous studies have evidenced that central arterial pressure was of greatest relevance for the assessment of the real load on cardiac performance.14 Since the augmentation of the aortic systolic pressure imposes larger afterload to the left ventricle, it determines a subsequent increase of the myocardial oxygen demand. However, peripheral and central systolic arterial pressures do not coincide. Pulse pressure increases in distal direction from the aorta to the brachial or radial artery. The phenomenon is known as pulse pressure amplification. It results from the lesser distance between the reflection sites and the peripheral arteries; consequently the earlier return of the backward wave in the cardiac cycle causes significant elevation of the peripheral systolic and pulse pressures. This pressure modification is especially pronounced in the young.15 Moreover, large interindividual variations of pulse pressure amplification are observed, i.e. large is the variability of the aortic pressure that corresponds to a given brachial value.16 For that reason peripheral systolic pressure turns out to be a less reliable measure of aortic pressure, consequently of cardiovascular risk. Here comes the advantage of applanation tonometry with the possibility for non-invasive estimation of central arterial pressure.
For better assessment of a bodily function it is always of benefit to apply an appropriate challenge in addition to its evaluation at rest. The active orthostatic test (OT) is a well-known physiological stimulus for the sympathetic division of the autonomic nervous system.17 The abrupt transition from supine to upright position provokes a significant redistribution of blood volume. Due to the effect of gravitational forces and the greater venous compliance a larger volume of blood remains in the lower portion of the body thus leading to decreased venous return and unloading of the arterial baroreceptors. The resulting higher sympathetic efferent discharge to the effectors heart and vascular smooth muscle cells as well as the parasympathetic withdrawal induces compensatory changes in heart rate and arterial pressure.18,19
The autonomic response to the orthostatic challenge might be evaluated through monitoring of heart rate variability (HRV) dynamics. The physiological variations in the cardiac cycle duration assessed through measurement of the RR-interval length fluctuations on the electrocardiogram (ECG) have been shown to reveal the cardiovascular autonomic influences.20,21 Fast Fourier Transformation analysis of the digitised ECG signal provides the short-term spectral HRV absolute powers: total power (TP), very low frequency component (VLF) (0–0.03 Hz), low frequency component (LF) (0.04–0.15 Hz) and high frequency component (HF) – 0.15–0.40 Hz. Still no consensus exists concerning the physiological significance of the above parameters. Most of the authors agree with the idea that the HF power reflects the parasympathetic modulation of cardiac function and it depends on respiration rate. The LF power is more controversial. Currently predominant are the views that LF power results from both sympathetic and parasympathetic influences. Slower and less periodic mechanisms like thermoregulation or certain hormonal effects determine the VLF HRV component. The derivative normalized LF (nu) and HF (nu) coefficients represent the relative proportion of LF and HF from TP (ignoring the VLF component) and are believed to avoid the impact of the large interindividual variation in the absolute TP typical for HRV. Thus LF (nu) is assumed to be a more reliable measure of the sympathetic modulation of HRV. The LF/HF ratio is assumed to represent the autonomic modulations of HRV.21
The aim of our study was to evaluate non-invasively using PWA the major central haemodynamic parameters at rest and under the effect of a challenge for the autonomic nervous system such as orthostasis. In addition, we considered the effect of age on the haemodynamic response to orthostatic stimulation and its relation to cardiovascular risk.
Methods
Twenty nine individuals were subjected to orthostatic testing. They were divided in two groups according to their age: group 60-YO (average age 59.8 ± 2 years, n = 16) and group 20-YO (average age 20 ± 0.3 years, n = 13). All examined subjects signed their informed consent to participate in the study, which was conducted according to the principles of the declaration of Helsinki.22 The experimental protocol was approved by the local Ethics Committee at our University.
The studied individuals rested for adaptation in supine position for 10 min.
The orthostatic test consisted of three experimental 5 min periods: baseline supine, upright and recovery supine position. Continuous electrocardiogram was recorded during the whole procedure by means of our analog-to-digital converter iCardio (IT Innovations Ltd.) for analysis of the heart rate response and for heart rate variability (HRV) evaluation.
At the end of each experimental period radial applanation tonometry was performed using the Sphygmocor device (AtCor Medical, Sidney, Australia). Brachial blood pressure was measured on the right arm using an Omron sphygmomanometer for calibration of the Sphygmocor device immediately before each radial pulse recording. Radial arterial pressures were recorded using the high-fidelity handheld probe. Only high quality measurements according to the recommendations of the AtCor Company were analysed. Central aortic pressures were assessed using the Sphygmocor software based on the generalised transfer function.
The parameters that were of utmost significance for the evaluation of the arterial wall status were the augmentation pressure and the augmentation index. The augmentation pressure was defined as the difference between the two systolic peaks: P2 − P1. The augmentation index (AIx) was calculated as
Short-term HRV was evaluated using the software packet iCardio. The digitised signal (256 s) from each of the 5 min experimental periods was subjected to Fast Fourier Transformation. All spectral indices of HRV were computed according to the Task Force recommendations.21 The LF, HF and TP absolute powers as well as the LF/HF ratio were transformed by natural logarithm to achieve normal distribution and to avoid skewing.23,24 The normalized LF (nu) and HF (nu) were calculated as well.
Statistical analysis was performed using the Data Analysis ToolPak of the Excel software and Vassar Statistics Software. The Wilcoxon–Mann–Whitney test, ANOVA and regression analysis were applied. The level of significance for p was assumed to be at least 0.05.
Results
The general characteristics and the dynamics of the monitored haemodynamic parameters are presented in Table 1.
Parameter | 60-YO | 20-YO | ||||
---|---|---|---|---|---|---|
Age (years) | 59.8 ± 2 | 20 ± 0.3 | ||||
BMI (kg/m2) | 27.7 ± 1* | 22.7 ± 0.6 | ||||
Baseline | Upright | Recovery | Baseline | Upright | Recovery | |
Heart rate (bpm) | 68 ± 2# | 75 ± 2* | 66 ± 2# | 68 ± 2# | 88 ± 3 | 65 ± 2# |
Radial SAP (mmHg) | 132 ± 6* | 128 ± 6 | 128 ± 5* | 116 ± 3 | 118 ± 4 | 115 ± 3 |
Radial DAP (mmHg) | 78 ± 5 | 79 ± 4 | 76 ± 3 | 72 ± 2# | 79 ± 2 | 73 ± 2# |
Radial PP (mmHg) | 54 ± 3* | 49 ± 3* | 52 ± 3* | 44 ± 3 | 40 ± 3 | 43 ± 3 |
Aortic SAP (mmHg) | 124 ± 6* | 119 ± 5* | 120 ± 5* | 99 ± 2 | 101 ± 3 | 98 ± 2 |
Aortic DAP (mmHg) | 77 ± 3 | 80 ± 4 | 77 ± 3 | 73 ± 2# | 80 ± 2 | 73 ± 2# |
Aortic PP (mmHg) | 47 ± 4*# | 38 ± 3* | 43 ± 3*# | 27 ± 2# | 22 ± 2 | 25 ± 2 |
AIx (%) | 33 ± 3* | 30 ± 2* | 35 ± 2* | −5 ± 4# | −16 ± 3 | −4 ± 4# |
AIx75 (%) | 30 ± 2* | 30 ± 2* | 31 ± 2* | −8 ± 4 | −9 ± 3 | −9 ± 4 |
Tr | 125 ± 4* | 128 ± 4* | 128 ± 3* | 153 ± 7 | 141 ± 4 | 148 ± 6 |
Data are presented as means ± SEM.
p at least 0.05 vs. 20-YO;
p at least 0.05 vs. upright position. BMI – body mass index; bpm – beats per minute; SAP – systolic arterial pressure; DAP – diastolic arterial pressure; PP – pulse pressure; AIx – augmentation index; AIx75 – augmentation index, corrected for heart rate 75 bpm.
General characteristics and dynamics of the non-invasive hemodynamic parameters during active orthostatic testing.
The 20-YO examinees were normal weighted (22.7 ± 0.6 kg/m2), while the 60-YO subjects were overweight (27.7 ± 1 kg/m2); their body mass index (BMI) was significantly higher (p < 0.05) as compared to the 20-YO.
Baseline heart rates were similar in the two groups; yet, the heart rate response to the upright posture differed markedly (Table 1). The 20-YO persons responded with a significantly elevated heart rate as compared to baseline and recovery values; the 60-YO individuals showed a minor heart rate increase, which was significantly lesser (75 ± 2 bpm, p < 0.05) than in the 20-YO group (88 ± 3 bpm).
The central and peripheral arterial pressure dynamics in response to the active orthostatic test is shown in Table 1 and Fig. 1. The baseline peripheral (brachial and radial) systolic pressure values were in the normal range in both groups. However, radial systolic (SAP) and pulse (PP) pressures were significantly higher in the 60-YO group as compared to the 20-YO persons during the three experimental periods. Aortic pressures differed significantly between groups as well. Aortic SAP and PP was significantly higher in the 60-YO individuals during the three experimental periods. Of particular interest was the markedly widened aortic pulse pressure (47 ± 4 mm Hg) in these persons at rest as compared to the same parameter in the 20-YO (27 ± 2 mmHg, p < 0.0001).
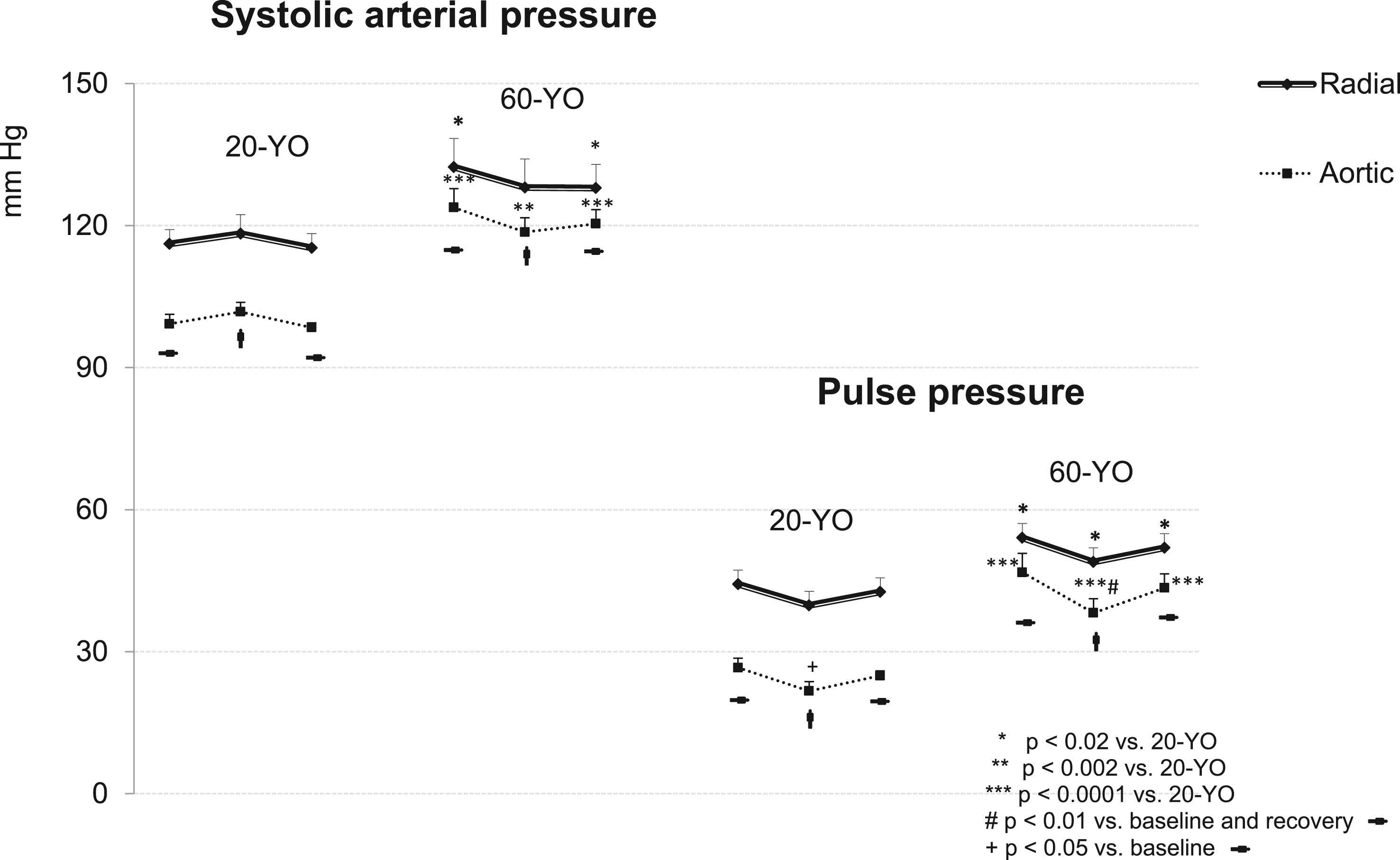
Dynamics of the radial and aortic (systolic and pulse) pressures during orthostatic testing. The significance of the differences between the 60-YO and 20-YO data was shown using the Wilcoxon–Mann–Whitney test; the in-group significance was shown using ANOVA. *p < 0.02; **p < 0.002; ***p < 0.0001 vs. 20-YO; #p < 0.01 vs. baseline and recovery; +p < 0.05 vs. baseline data.
Similarly to heart rate, blood pressure response pattern to the orthostatic challenge differed between groups. Moderate fall of the radial and aortic systolic pressure was observed in the 60-YO group during the upright period (though marginally significant only for the aortic pressure, p < 0.055). On the contrary, the 20-YO individuals did not show any noteworthy change in the radial or aortic SAP. The upright standing caused a trend for radial PP decline in both groups. The aortic PP reduction was more pronounced. Aortic PP was reduced significantly in the 60-YO as compared to the baseline and recovery resting levels; in the 20-YO subjects PP reduction was statistically significant only as compared to its initial level.
Diastolic pressure dynamics is shown in Fig. 2. In contrast to systolic and pulse pressures no difference between radial and aortic diastolic values was observed in both groups. Diastolic pressures were higher in the 60-YO as compared to the 20-YO group at resting conditions; yet, the difference did not reach statistical significance probably because of the large intra-group variations. The 20-YO subjects responded to the orthostatic challenge with a significant rise of both radial and aortic diastolic pressure (p < 0.002 vs. baseline and recovery). On the contrary, the 60-YO individuals reacted with an only minor elevation of diastolic pressure at the end of the upright standing period.
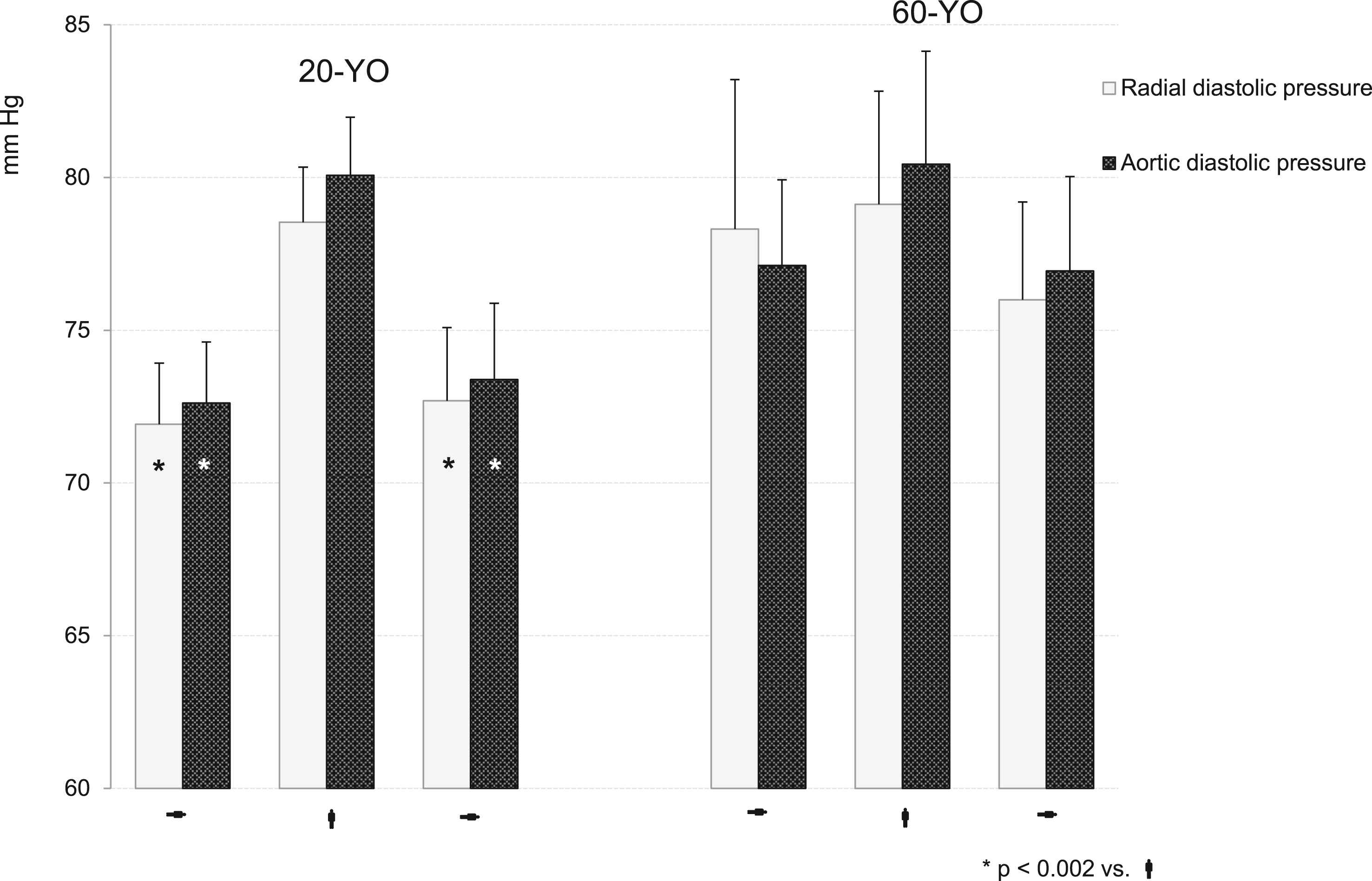
Dynamics of the radial and aortic diastolic pressures during orthostatic testing. The in-group significance was shown using ANOVA. *p < 0.002 vs. active standing.
The aortic pulse waveform in the 60-YO was typically of A-type during the three experimental periods. Both augmentation indices AIx and AIx75 were significantly higher as compared to the 20-YO individuals (Fig. 3, p < 0.0001). AIx showed a trend for reduction under the effect of orthostatic stimulation, while no change was observed in AIx75. The pulse waveform in the 20-YO was of C-type with negative augmentation pressure and augmentation indices. Both AIx and AIx75 grew more negative in response to the orthostatic challenge; however, only AIx reduction was statistically significant (Fig. 3, p < 0.01).

Dynamics of AIx and AIx75 during orthostatic testing. The significance of the differences between the adult and young adult data was shown using the Wilcoxon–Mann–Whitney test; the in-group significance was shown using ANOVA. *p < 0.0001 vs. 20-YO supine; **p < 0.00001 vs. 20-YO supine; #p < 0.01 vs. active standing.
Time to return was significantly longer in the 20-YO group during the three experimental periods (Fig. 4). It showed a trend of shortening in this group as a response to the orthostatic challenge, while in the 60-YO it did not change at all.
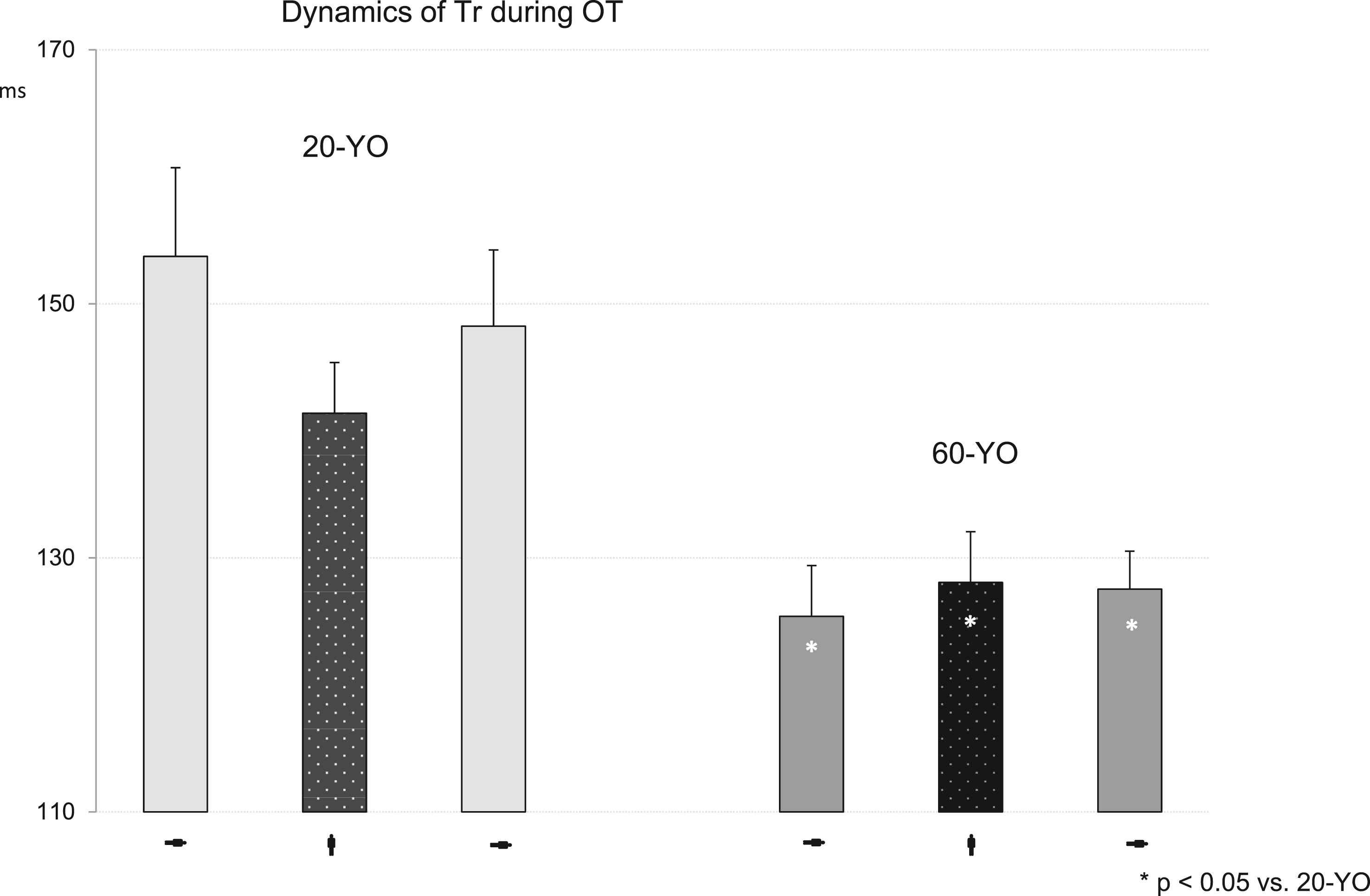
Dynamics of Tr during orthostatic testing. The significance of the differences between the adult and young adult data was shown using the Wilcoxon–Mann–Whitney test. *p < 0.05 vs. 20-YO.
The HRV dynamics during active orthostatic testing is presented in Table 2 and Fig. 5. The absolute powers of HRV – TP, LF and HF components – were significantly lower in the 60-YO group as compared to the 20-YO individuals during all the three experimental periods (p < 0.02). The normalized LF (nu) and HF (nu) indices and the ln LF/HF ratio were lower in the 60-YO group only during upright standing (p < 0.05). The within group differences were more pronounced in the 20-YO group. The absolute LF power rose significantly in response to active standing (p < 0.05). The absolute HF power was markedly reduced during the upright period (p < 0.05); it rose to an even higher than the baseline level value during recovery (p < 0.01). The normalized indices and the ln LF/HF were significantly higher during the active standing period as compared to both baseline and recovery periods (p < 0.01). HRV indices were subjected to minor modifications in the 60-YO group. Only the normalized indices and the ln LF/HF showed statistically significant changes during the recovery period as compared to the active standing (p < 0.05).
Parameter | 60-YO | 20-YO | ||||
---|---|---|---|---|---|---|
Baseline | Upright | Recovery | Baseline | Upright | Recovery | |
ln LF (ln ms2) | 5.9 ± 0.3** | 6 ± 0.3** | 5.8 ± 0.3** | 7.4 ± 0.2 | 7.9 ± 0.2# | 7.7 ± 0.1 |
ln HF (ln ms2) | 6 ± 0.4** | 6 ± 0.3** | 6 ± 0.4** | 7.4 ± 0.3++ | 6.7 ± 0.3#++ | 8.2 ± 0.3 |
ln TP (ln ms2) | 6.7 ± 0.3** | 6.5 ± 0.3** | 6.7 ± 0.4** | 8.2 ± 0.2++ | 8.2 ± 0.2+ | 8.8 ± 0.2 |
LF (nu) | 48 ± 5 | 59 ± 5*+ | 44 ± 5 | 51 ± 5 | 74 ± 5##++ | 41 ± 5 |
HF (nu) | 52 ± 5 | 41 ± 5*+ | 56 ± 5 | 49 ± 5 | 26 ± 5##++ | 59 ± 5 |
ln LF/HF | −0.1 ± 0.2 | 0.4 ± 0.2*+ | −0.3 ± 0.2 | 0.07 ± 0.2 | 1.2 ± 0.2##++ | −0.4 ± 0.2 |
Data are presented as means ± SEM.
p < 0.02;
p < 0.05 vs. 20-YO;
p < 0.05,
p < 0.01 vs. baseline;
p < 0.05,
p < 0.01 vs. recovery.
Heart rate variability dynamics during active orthostatic testing.
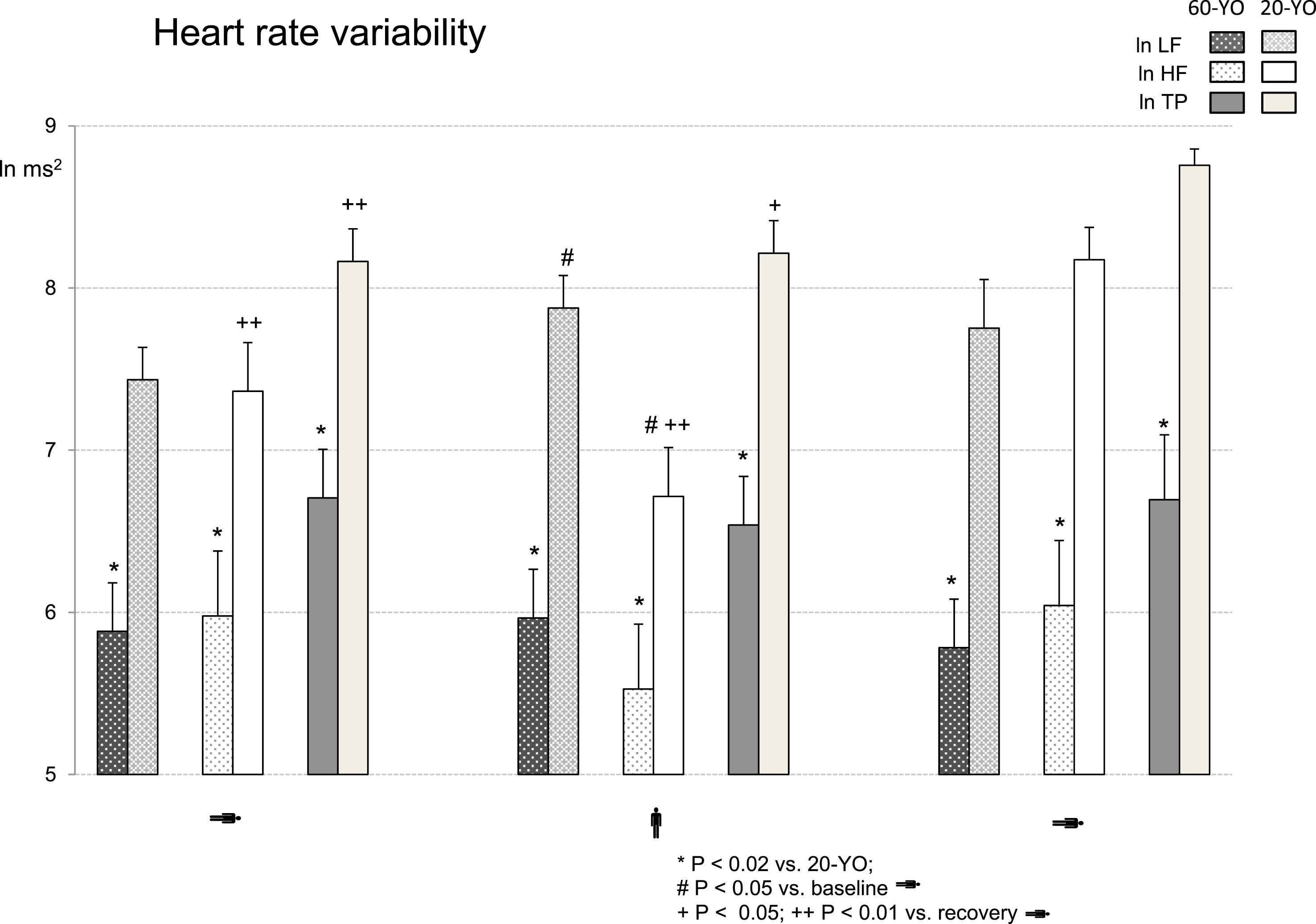
Dynamics of HRV absolute powers during orthostatic testing. Low frequency (LF), high frequency (HF) and total power (TP) of HRV are presented as natural logarithms. The significance of the differences between the 60-YO and 20-YO data was shown using the Wilcoxon–Mann–Whitney test; the in-group significance was shown using ANOVA. *p < 0.02 vs. 20-YO; #p < 0.05 vs. baseline; +p < 0.05; ++p < 0.01 vs. recovery.
Discussion
Active orthostatic test
The autonomic response to the circulating blood volume redistribution as a result of postural changes has been the subject of numerous studies in the past. The role of various reflex mechanisms, i.e. cardiopulmonary, venoarteriolar, the key baroreflex afferentation, the resulting parasympathetic withdrawal and increased sympathetic outflow to the effectors heart and vascular smooth muscle were systematically studied.25–28 On the contrary, comparatively few data exist concerning the central haemodynamic alterations associated with the orthostatic challenge, especially in response to the active orthostatic test. The first documented simultaneous central and peripheral arterial pressure modifications during postural challenge in healthy human volunteers were reported using invasive measurements in 1955.29 More recently non-invasive techniques were introduced for study of the impact of postural changes on central haemodynamics. However, most of the authors utilised the tilt test, passive leg raising or sit-to-stand test.30–33 In general, it is supposed that the tilt test provokes more exaggerated reactions since the “muscle pump” compensation is absent. Various putative mechanisms were suggested to be involved in the response to the active standing test that were absent during the passive tilt: emphasis was placed on the primary role of the muscle pump for the early compensation of venous return as well as on the mismatch between cardiac output and vascular compliance responsible for the initial hypotension, especially in the elderly.34 According to the traditions in our laboratory we have focused on the haemodynamics of the active standing test. We assumed the active standing test was more informative since the subsequent haemodynamic and autonomic alterations resulted from the involvement of pure physiological mechanisms.
Our objectives to apply the same experimental protocol to individuals of two age groups were the typical modifications of the vascular wall and the age related autonomic function changes. We supposed that ageing might interfere with the response mechanisms and we were interested to compare the autonomic and the haemodynamic response pattern between the two age groups.
Heart rate
We found of interest the significantly minor heart rate elevation at the end of the 5th minute of active standing in the 60-YO group as compared to the 20-YO individuals (Table 1). One plausible explanation might be related to alterations in the signalling mechanisms of the pacemaker cells themselves35 or in the receptor sensitivity36 in the ageing heart. We found as more relevant another possibility: an attenuated parasympathetic arterial baroreflex might explain the less pronounced heart rate response to orthostasis. In a recent review regarding the effect of ageing on arterial baroreflex the idea of diminished reflex interbeat interval modifications in response to blood pressure changes was discussed.37 Arterial stiffening, oxidative stress and decreased cardiac cholinergic responsiveness were proposed as putative mechanisms. Additional evidence in support to the baroreflex attenuation will be presented further in the discussion.
Arterial pressure
Applanation tonometry provided accurate and detailed information about the central cardiovascular haemodynamics during the active orthostatic testing. The baseline peripheral systolic and pulse pressure values in both groups were in the normal range according to the ESH and ESC guidelines.38 However, they were significantly higher in the 60-YO as compared to the 20-YO individuals (Table 1, Fig. 1). We interpreted this finding as a further manifestation of the physiological stiffening and decreased arterial distensibility with ageing.39,40 This assumption was particularly supported by the wide aortic pulse pressure (more than 40 mmHg according to the Mayo clinic criteria).
The difference in the pressure response to the orthostatic challenge might also be associated with the increased arterial stiffness in the 60-YO. The well-defined trend for brachial and aortic systolic pressure decrease in the 60-YO on the 5th minute of active standing upright supported this supposition (Fig. 1). Even more convincing was the radial pulse pressure and especially the aortic pulse pressure narrowing. In contrast, the 20-YO individuals responded with lack of change in the systolic pressure at the end of the active standing upright and a less pronounced lowering of pulse pressure. We assumed this difference between groups also resulted from the less efficient compensatory reaction to the blood retention in the lower portion of the body. The stiffening of the large arteries was already suggested as an underlying mechanism for decreased carotid and aortic baroreceptor sensitivity in the 60-YO individuals. The diminished capacity for stretch or relaxation of the stiff arterial wall was proposed to result in lesser stimulation or deactivation of the mechanosensitive channels in the barosensitive carotid and aortic areas.41,42 As a consequence to the altered baroreceptor afferentation in the 60-YO the reflexive sympathetic efferent input to the heart was lesser; therefore, the insignificant heart rate elevation and the significant reduction of aortic pulse pressure. On the contrary, the unloading of the arterial baroreceptors produced the adequate sympathetic chronotropic and inotropic stimulation to the heart in the 20-YO individuals. The optimal haemodynamic compensation resulted in an only minor decrease of the aortic pulse pressure; both peripheral and aortic systolic pressures did not change at all.
In summary, we believe that the specific pattern of systolic and pulse pressure response to the orthostatic challenge in the two experimental groups was an additional evidence in favour of the arterial stiffness causative significance. In general, the BMI difference between groups might also be considered as underlying factor for the pressure differences. However, we regard this supposition as less plausible. On the one hand the BMI difference was not considerable, the 60-YO persons were overweight, not obese. On the other hand, the systolic and pulse pressure response pattern to the active standing task spoke against such a supposition. The existing literature data show inverse relationship between body mass and orthostatism. Orthostatic hypotension is related to lower body mass or is observed in obese individuals after body mass reduction.43
In contrast to systolic and pulse pressure resting diastolic pressure in both groups did not differ markedly between the aorta and the peripheral medium sized arteries such as the brachial or radial ones (Fig. 2). This phenomenon is well-known and it is attributed to the comparatively large diameter of the arteries up to the radial artery and, therefore, the small resistance they exert to blood flow.44,45 The 60-YO individuals did not respond to the orthostatic challenge with any change in both radial and aortic diastolic pressure, while the 20-YO persons reacted with a pronounced diastolic pressure elevation. This finding is in harmony with the reported inverse correlation between carotid artery stiffness and sympathetic baroreflex in the elderly.46 Our supposition for reduced arterial baroreflex efficiency and decreased sympathetic efferent discharge to the resistive arterioles in our 60-YO individuals is supported by data that showed impaired baroreflex and reduced muscle sympathetic nerve activity through direct recording in the elderly.47
Heart rate variability
We analysed HRV for non-invasive evaluation of the autonomic influences on the cardiovascular system in response to the active orthostatic test (Table 2, Fig. 5). The significantly lower absolute powers of HRV observed in the elderly during the three experimental periods were in agreement with studies showing age dependence and gradual reduction of HRV with ageing.48–50 The HRV data supported our assumptions for reduced baroreceptor sensitivity and the ensuing less efficient sympathetic stimulation in the 60-YO individuals. No significant alteration in the monitored HRV parameters in response to the active upright position was observed in this group. The lack of significant elevation of the LF absolute power, LF (nu) and ln LF/HF ratio during the upright challenge was in parallel with the trend for systolic pressure decrease and the negligible diastolic pressure changes. On the contrary, the significant elevation of the absolute and normalized LF power and of the ln LF/HF ratio in response to the active upright standing in the 20-YO individuals (both as compared to their baseline values as well as to the values in the 60-YO group) might be interpreted as manifestation of an effective arterial baroreceptor regulation of arterial pressure and of the potent stimulation of the cardiovascular sympathetic efferent activity. In addition, significant parasympathetic withdrawal during the upright period was observed in the 20-YO group as shown by the marked fall of the absolute and normalized HF HRV, which was less pronounced in the 60-YO individuals. The pattern of cardiovascular reactivity in the two groups during the recovery phase differed as well. The prompt and marked shift in the LF and HF HRV values (both absolute and normalized) in the young examinees was an evidence for the significant vagal activation and sympathetic withdrawal during the recovery supine position. Even more, during recovery the absolute HF and TP HRV parameters reached superior values than the baseline as an expression of the more powerful parasympathetic efferent modulation upon the sinus node pacemaker function.
Augmentation indices
The positive indices AIx and AIx75 in the 60-YO at baseline were in harmony with the data from many previous studies as well as with our above findings (Table 1, Fig. 3).4,5,41 They presented an additional evidence for the stiffened cushioning arteries and the early return of the reflected wave in the 60-YO examinees.8,44 On the contrary, the normal arterial distensibility and pulse wave velocity in the 20-YO persons determined the baseline negative values of the augmentation pressure and the two augmentation indices due to the late return of the reflected wave in diastole.
The dynamics of the augmentation indices in response to the orthostatic challenge corresponded to the different haemodynamic profiles in the two groups. In the 20-YO subjects AIx dropped significantly as compared to the baseline and recovery data, while AIx75 reduction was minor and insignificant. We attributed this dissociation to the significant reflex tachycardia in the young persons, which determined the relative delay of the pulse wave return in respect to the shortened cardiac cycle. Our data coincide completely with the described highly significant inverse negative correlation between the magnitude of the augmentation index and heart rate.51 The lack of change in AIx75 might be considered as an additional proof for the absence of short-term alterations in arterial stiffness in response to active standing in the 20-YO group. This finding is supported by recent data for lack of autonomic modification of the heart rate and pressure adjusted pulse wave velocity in young and healthy individuals.52
On the contrary, AIx decreased insignificantly at the end of the standing period in the 60-YO group and remained positive. We supposed that on the background of the stiffened arterial vessels the minor heart rate acceleration produced a negligible relative delay in the timing of the reflected wave. Accordingly, the correction of AIx for the standard heart rate of 75 bpm completely abolished the augmentation index modification.
In general, the existing data concerning the AIx changes in response to orthostatic challenge vary and they are to some extent contradictory. Certain authors reported decrease of AIx, others – dependency of the AIx change on the degree of tilt.30,53,54 Recent study presented evidence for dissociation between the effects of passive head up tilt and lower body negative pressure application on AIx. The authors reported that only the huge change in aortic haemodynamic in response to the lower body negative pressure stimulation resulted in AIx decrease while passive head up tilt was inefficient.55 These divergent findings probably resulted from the varying protocols, methods of pulse wave analysis (applanation tonometry or oscillometric methods), age, etc. We believe our AIx data confirmed the idea for age-specific mechanisms in the body response to active orthostasis.
Time to return of reflected wave (Tr)
Shortening of Tr is an evidence of arterial stiffening as it is a consequence to the elevated forward and backward PWV.56
As it might be expected, this parameter was significantly lesser at the end of all three experimental periods in the 60-YO individuals as compared to the 20-YO group (Fig. 4). Moreover, Tr remained unchanged under the effect of active standing in this group in confirmation of the above augmentation index data. It was definitely shown that peripheral vascular resistance did not influence wave reflection directly. However, acute transitory modifications in arterial stiffness were suggested to be pressure induced.12 Similar data regarding the effect of vasoactive agents on the augmentation index have been reported as well.57 Therefore, the lack of change in diastolic and, correspondingly, in mean arterial pressure were in accordance with the Tr dynamics in the 60-YO examinees. On the contrary, the active standing provoked a slight trend towards earlier reflected wave return in the 20-YO persons, yet the difference did not reach statistical significance. We believe that the marked diastolic pressure elevation in the 20-YO group at the end of the standing period was the putative cause for Tr shortening.
In conclusion, we expect our findings will contribute to understanding the haemodynamics of orthostasis in general. In addition, certain age-specific patterns of cardiovascular reactivity were shown. These differences were attributed to the natural structural alterations in the arterial wall that accompany ageing: the arterial stiffening, the consequent changes in the arterial distensibility and pulse wave velocity, the decreased baroreflex sensitivity (BRS) and the lesser efficiency of the compensatory response to orthostasis in the older subjects. This assumption was confirmed by the HRV analysis that showed minor sympathetic activation in the 60-YO individuals in response to the orthostatic challenge as compared to the autonomic adjustments in the 20-YO group. The imperfect adaptation of the haemodynamics to the orthostatic challenge in the 60-YO group might be observed as one additional facet of the elevated cardiovascular risk typical for ageing.58
Applanation tonometry and routine PWA have not yet found their appropriate status in general cardiological practice. Meanwhile, simply adding blood pressure measurement in upright position to the conventional procedure in the office plus thorough analysis of pulse pressure response might be of relevance for cardiovascular risk screening.
Limitation of the study
Our studies would have benefited from the direct measurement of the carotid-femoral pulse wave velocity as a further confirmation of the arterial stiffening with age. We have avoided this method only because of certain ethical issues related to the procedure.
Conflict of interest statement
There are no conflicts of interest related to the current study.
Acknowledgement
This study was partially supported by the
References
Cite this article
TY - JOUR AU - Rene Mileva-Popova AU - Nikolay Stoynev AU - Nina Belova PY - 2017 DA - 2017/07/30 TI - Applanation tonometry for evaluation of the haemodynamic response to the active orthostatic test JO - Artery Research SP - 72 EP - 82 VL - 19 IS - C SN - 1876-4401 UR - https://doi.org/10.1016/j.artres.2017.07.001 DO - 10.1016/j.artres.2017.07.001 ID - Mileva-Popova2017 ER -