The role of “semicarbazide-sensitive amine oxidase” in the arterial wall
- DOI
- 10.1016/j.artres.2009.10.002How to use a DOI?
- Keywords
- Semicarbazide-sensitive amine oxidase; Extracellular matrix; Crosslinks; Vascular smooth muscle cells; Arterial stiffness
- Abstract
“Semicarbazide-sensitive amine oxidase” (SSAO) metabolizes primary amines into aldehydes, hydrogen peroxide and ammonia and is largely expressed in adipocytes, lymphatic endothelial cells and vascular smooth muscle cells (VSMCs). In vitro SSAO expression and activity increase during VSMC differentiation and SSAO participates in glucose uptake but its exact role in the vascular wall is unclear. Nevertheless, we think that SSAO could modulate VSMC differentiation, as it does in adipocytes. Previous work suggested that SSAO could contribute to vascular remodelling observed in age-related pathologies as plasma levels increase in several cardiovascular diseases and in diabetes. In such cases, SSAO may play a detrimental role by inducing unwanted cross-linking of extracellular matrix proteins and could thus be an interesting target to modulate arterial stiffening. It may also participate in LDL oxidation and inflammation.
A physiological role for SSAO in vascular development and cross-linking of elastin has been proposed, but is not yet established. Our studies on SSAO knockout mice revealed no anomalies in insoluble elastin content, arterial elasticity or vasomotor tone. In growing rats treated with semicarbazide (SCZ), an SSAO inhibitor, or with β-aminopropionitrile (BAPN), a lysyl oxidase (LOX) inhibitor, we confirmed that LOX was the most important enzyme implicated in elastin cross-linking during arterial maturation, but in absence of specific inhibitors, we cannot formally exclude a minor role for SSAO in this process. Specific effects of SSAO inhibition could be an increase in the production of various extracellular matrix proteins, possibly related to the effect of SSAO on VSMC differentiation.
- Copyright
- © 2009 Association for Research into Arterial Structure and Physiology. Published by Elsevier B.V.All rights reserved.
- Open Access
- This is an open access article distributed under the CC BY-NC license.
Semicarbazide-sensitive amine oxidase (SSAO) was originally characterized by its activity in tissue and plasma and its sensitivity to carbonyl compounds, such as semicarbazide (SCZ). Only ten years ago, SSAO was cloned in different species and a high inter-species amino acid homology was observed. Cloning has facilitated the exploration of its functions, which nevertheless still remain unclear. In fact, SSAO also exists as a soluble form whose activity is increased in the serum in patients with diabetes1–3 and cardiovascular diseases, including congestive heart failure,4 cerebrovascular diseases5 and atherosclerosis.6 SSAO activity also correlates positively with body mass index.2 Serum levels of SSAO are also increased during inflammation7 and other diseases (review, ref.8).
SSAO, also called vascular adhesion protein 1 (VAP-1), is an ecto-enzyme with a long extracellular domain containing the catalytic site, which requires a topaquinone and one copper atom as cofactors. SSAO metabolizes primary amines into aldehydes, ammonia and hydrogen peroxide. The physiological substrates for SSAO still remain largely unknown. However, SSAO can metabolize methylamine (generated from adrenaline, creatine, creatinine and choline metabolism, food or cigarette smoke) but also aminoacetone (generated from glycine and threonine).9 The crystal structure of SSAO members clearly indicates that SSAO can also interact with free amino groups in proteins and aminosugars.10–12
Tissue and plasma SSAO activity is much higher in humans than in rodents. SSAO expression occurs early in development13 and continues throughout life in many cell types but mainly in adipocytes, endothelial cells and vascular smooth muscle cells (VSMCs). The origin of soluble SSAO is beginning to be elucidated. SSAO release is increased by TNFα via MMP activity in adipocytes14 and regulated by β-adrenergic agonists and insulin,15,16 the latter explaining the plasma SSAO increase in diabetes. Moreover, different transgenic mouse models have shown that adipocytes,17 but also vascular endothelial cells17 and VSMCs18 can be major sources of plasma SSAO.
It was first suspected that SSAO could be a source of toxicity for the arterial wall via aliphatic amine metabolism. For example, allylamine, which can be oxidized by SSAO, was shown to induce necrotic and fibrous lesions in myocardial and vascular tissues, modifications observed in atherosclerosis, via acrolein (generated from allylamine by SSAO) and H2O2 production.9 Regarding the end products formed by SSAO, aldehydes and hydrogen peroxide can increase protein cross-linkage and oxidative stress, two phenomena involved in aging. It is interesting to note that methylamine or aminoacetone deamination by SSAO generates respectively formaldehyde and methylglyoxal and increases the formation of advanced glycation end products (AGEs).17,19–21 AGEs can induce an increase in arterial stiffness in diabetes, hypertension and aging.22,23 The interaction of AGEs with their receptors (RAGE) induces the expression of pro-inflammatory mediators. An important role is attributed to RAGEs in vascular diseases in the literature.24 Some therapeutic agents are able to inhibit AGE formation, such as aminoguanidine that is also an SSAO inhibitor.25 It has been shown that diamine oxidase (another topaquinone-containing amine oxidase) can catalyze endothelial cell-mediated LDL oxidation.26 The increased SSAO activity in plasma during diabetes mellitus could thus accelerate the development of the degenerative complications of this pathology.9 The combination of both SSAO end products may thus participate in the formation of advanced glycation end products (AGEs) and in LDL (low density lipoprotein) oxidation and be implicated in atherogenesis. Furthermore, endothelial SSAO participates in leukocyte trafficking and transmigration during inflammation and we demonstrated that methylamine-SSAO activation induces expression of endothelial E and P-selectins, one step of the adhesive cascade, via generation of its end products.27
Soluble SSAO incubated with methylamine on rat and human VSMCs induced cell toxicity, mediated by the end products generated. It activated apoptosis in A7r5 cells, as detected by chromatin condensation, Caspase-3 activation, PARP cleavage and cytochrome c release to cytosol due to formaldehyde, rather than H2O2.28 Methylamine treatment of a smooth muscle cell line stably transfected with SSAO also induced a dose- and time-dependent cytotoxic effect involving the tumour suppressor protein p53, increased PUMA-alpha expression with a decrease in mitochondrial Bcl2 family proteins and activation of caspases.29
Taken together, all these results suggest that increased soluble and tissue SSAO activity in pathological conditions, could contribute to vascular damage. Thus, it is of potential clinical interest to better understand the role of SSAO in human physiology and physiopathology and to develop specific SSAO antagonists.
Before starting my PhD, SSAO was already known to be involved in amine-stimulated glucose transport in adipocytes,30 cell adhesion, lymphocyte trafficking and granulocyte extravasation in endothelial cells from lymphatic vessels.27 Our work has been mainly aimed at investigating a possible physiological role of SSAO during vascular growth and maturation.
Personal contributions
SSAO, cell differentiation and glucose transport in vitro
In our laboratory, SSAO was cloned in a mouse adipose cell line where its expression is induced during adipose maturation.31 H2O2, known as an insulin-mimicking molecule in adipocytes, was largely responsible for the SSAO-promoting effect on adipose cell differentiation.32 Moreover, SSAO expression was shown to be down-regulated by TNFα31,32 and AMPc31 in adipocytes. Others found that endothelial SSAO could be up-regulated by TNFα but also by other inflammatory cytokines (IL-1, IL4, Interferon γ), and LPS.33
In parallel, we were also interested in SSAO functions in the arterial wall. In physiological conditions, SSAO is largely expressed in the media from large vessels in vivo, which contains mainly SMCs.34,35 It was also shown that both VSMCs and non-vascular SMCs in culture expressed SSAO.36,37 We have shown that re-differentiation of cultured VSMCs in a serum-free medium supplemented with growth factors induced expression and activity of SSAO, in parallel with other differentiation markers.38 It is possible that glucose and H2O2 may act as a signal modulating the expression of genes coding for differentiation markers and ECM proteins. Even though SSAO activation promotes adipose cell differentiation through H2O2,32 no direct argument has yet shown the implication of SSAO in VSMC maturation. Nevertheless, in some forms of aneurysm, the expression of SSAO and smooth muscle myosin heavy chain (a marker of VSMC differentiation) are both markedly decreased at the aneurysmal site where elastic fibres are disorganised.39 In contrast, SSAO is expressed along the elastic lamellae in the non-affected zone.40 This is in accord with our in vitro results. The vascular system, adipose tissue, and smooth muscle cells express SSAO early during embryonic development suggesting that SSAO could be involved in the differentiation process (as in tissue vascularisation) during this period.13
We have also shown in vitro that H2O2, generated via methylamine oxidation by SSAO, increases glucose transport and membrane accumulation of GLUT1.38 An association between a GLUT mutation and aneurysm formation has recently been shown that might be explained by an overproduction of TGFβ and VSMC dedifferentiation.41,42 Thus these results reinforce the idea that SSAO could be implicated in VSMC differentiation. In an in vivo rat model of cerebral aneurysms, it has recently been shown that a decrease in SSAO activity and expression, associated with SMC dedifferentiation, is strongly and independently correlated with elastic laminar thinning during the development of intracranial aneurysms.39 The authors suggested that VSMC dedifferentiation induced by hemodynamic stress could impair elastic lamellar organization through a decrease in elastin content and a possible SSAO-related elastin cross-link deficiency.
Possible role of SSAO in organization and maturation of arterial ECM
The maturation and structure of elastic fibres and collagen depends on the formation of intra- and intermolecular crosslinks, a physiological process which occurs in development and growth. This process strongly influences elasticity and resistance of large arteries. Lysyl oxidase (LOX), an enzyme belonging to the same family as SSAO, is the major enzyme involved in this pathway (review, ref.43). Inactivation of the LOX gene leads to perinatal death and to aortic aneurysm formation.44 Langford et al.45 have suggested that SSAO could play an important role in arterial wall organization as they showed that chronic inhibition of SSAO in young rats was associated with aortic damage and dilatation, with elastic lamellar disorganization. In vitro experiments confirmed that SSAO inhibition produced aberrations in collagen and elastin deposition by heart SMCs.46 Furthermore, Gokturk et al.18 have developed a transgenic murine model over-expressing human VAP-1 in VSMCs. These mice presented an elevated pulse pressure, together with an abnormal elastic lamellar structure in the aorta, suggesting increased rigidity of large arteries as a result of an elevated SSAO activity, as well as a possible physiologic role for SSAO in elastic fibre maturation. In addition, as mentioned above, a strong decrease in SSAO was observed in aortic and cerebral aneurysms and a co-localization was found between SSAO and elastic fibres in the peripheral unaffected zones.39 All these results provide arguments to support a role for SSAO in the synthesis, maturation and/or organization of ECM proteins, and elastin in particular.
An in vivo rat model of pharmacological SSAO inhibition
Based on these observations, we examined the consequences of chronic inhibition of SSAO by SCZ during the period of rapid growth in the Sprague Dawley rat on the functional, histological and biochemical properties of large arteries.47In vivo, distensibility was not modified whereas the Einc/wall stress curves showed an increased arterial stiffness in response to SCZ. In vitro experiments revealed a significantly decreased rupture pressure in carotid arteries from SCZ-treated rats compared to controls. This result indicated that arterial fragility is induced by SCZ, which was accompanied by a diminution in insoluble, crosslinked elastin and alterations of elastic lamellae. Elastic lamellar structure was modified by the presence of peripheral deposits of dense globular masses, supposed to be composed of non-crosslinked elastin and proteoglycans. Because these effects were similar to those observed after pharmacological inhibition of LOX by BAPN,48,49 we measured LOX activity in SCZ-treated rats. In contrast to the results of Langford et al.,45 we found a significant (40%) inhibition of LOX activity induced by SCZ. Thus, it was not possible to know whether the effects induced by SCZ were due to SSAO or to LOX inhibition. In SCZ-treated rats, the medial thickness was also increased in association with an increased weight of extracellular proteins other than insoluble elastin and collagen.
An SSAO knockout mice model
In order to avoid the confounding effects of SCZ-induced LOX inhibition, we examined the vascular phenotype of SSAO deficient mice.50In vivo, SSAO knockout mice presented a decrease in carotid distensibility in association with an increase in carotid diameter compared to WT mice. In SSAO knockout mice, arterial morphology was normal, there was no reduction in crosslinked elastin or in total collagen, muscular tone was not modified and elastic modulus was unchanged. These results could not be explained by a compensatory increased in LOX activity in the KO mice. We thus could not confirm the hypothesis of a role for SSAO in cross-link formation, at least during development and growth in the mouse.
It has been proposed that H2O2 generated by SSAO could increase vascular tone and that inhibition of SSAO could participate in vasodilatory effects induced by hydralazine in the rat.51–53 But these results diverge from those obtained by Coklin et al.54 on human left internal mammary artery segments, where methylamine induced vasodilatation via formaldehyde and H2O2 production. However, in our SSAO knockout mouse model, the pharmacological profile of isolated mesenteric and carotid arteries was not modified compared to wild type mice, implying no major VSMC or endothelial dysfunction in the absence of SSAO, at least in mice.50
Comparison of the roles of LOX and SSAO in arterial extracellular matrix
To attempt to discriminate between SCZ effects on the ECM that were due to LOX inhibition from those due to SSAO inhibition, we used high and low equimolar doses of BAPN (a specific LOX inhibitor) and SCZ to treat Brown-Norway (BN) rats during the rapid growth period.55 The BN rat presents a rare arterial phenotype implicating the elastic fibre network (Large numbers of defects in the internal elastic Lamellae (IEL), a deficit in aortic insoluble elastin and a lower LOX activity compared to other strains). This strain was chosen, rather than the previously used Sprague Dawley, because IEL rupture is particularly sensitive to compounds affecting elastin and collagen cross-linking and is precisely quantifiable.48,56 The BN is thus is a good model in which to carry out such a study. Our results showed that SCZ was as efficient as BAPN in inhibiting LOX activity but SCZ inhibited SSAO activity in aorta far more than BAPN. Both inhibitors decreased arterial rupture pressure and insoluble elastin content and increased collagen solubility and IEL rupture, but SCZ was more potent. Thus either these effects are all mediated by LOX, SCZ being a more effective LOX inhibitor than BAPN in our conditions, or SSAO acts similarly to and in synergy with LOX on the extracellular matrix, but playing a minor role. We must await the availability of more specific inhibitors to definitely settle the question. However, aortic weight was increased only by the high dose of SCZ, mainly due to increased ECM proteins other than insoluble elastin, and to a lesser extent by increased collagen, suggesting that this may be a specific effect of SSAO inhibition. This may be linked to a role for SSAO in VSMC differentiation.
The main animal and cell models that may help to understand the role of SSAO in the arterial wall are summarized in Table 1 and the various mechanisms proposed are schematized in Fig. 1.
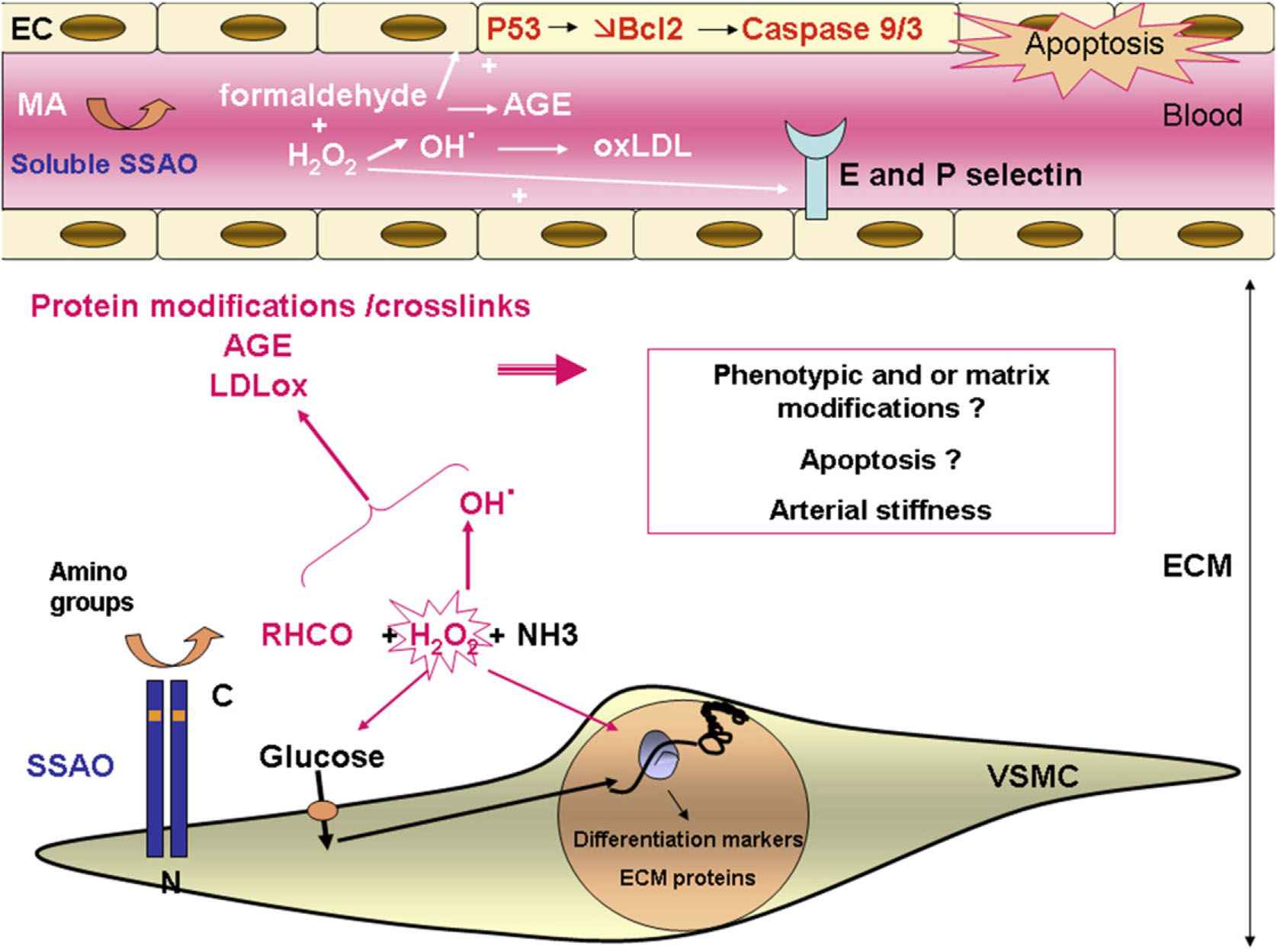
Summary of possible roles of SSAO in the vascular wall. SSAO is expressed at the plasma membrane of differentiated VSMCs with its active site located in the extracellular space, where it transforms amino groups from primary amines or within proteins into aldehydes (RHCO), hydrogen peroxide (H2O2) and ammonia (NH3). SSAO activates amine-stimulated glucose transport partly through H2O2. Glucose and H2O2 may act as a signal modulating the expression of genes coding for differentiation markers and ECM proteins. H2O2 is a source of hydroxyl radicals that can combine with aldehydes and participate in protein modifications, in the formation of crosslinks and advanced glycated end products (AGE) and in oxidation of low density lipoproteins (ox LDL). These events could lead to modification of VSMC phenotype, organization of ECM, apoptosis and/or arterial stiffness. SSAO has also been shown to be expressed by endothelial cells (EC) during inflammation and can up-regulate E and P selectin expression. SSAO is also present in the serum where the end products generated from methylamine (MA) could also participate in LDL oxidation, AGE formation and apoptosis induction via activation of P53 and a decrease in Bcl2 leading to activation of caspase 9 and 3. Thus, SSAO might play a role in vascular wall physiopathology, especially in atherosclerosis.
Genetically modified mice | Pharmacological SSAO inhibition in growing rats | Cell models |
---|---|---|
|
|
VSMC differentiation38
|
SMCs from neonatal rat46
|
||
|
|
Effect of soluble SSAO on VSMCs28
|
Mice over-expressing hSSAO in endothelial cells17
|
|
Comparison of the different animal and cell models used to elucidate the role of SSAO in the arterial wall.
Conclusion
Previous work has provided several lines of evidence that SSAO could contribute to vascular remodelling observed in age-related pathologies (especially cardiovascular diseases and diabetes) by inducing unwanted cross-linking of extracellular matrix proteins and participating in LDL oxidation and inflammation. SSAO could thus be an interesting therapeutic target to modulate, in particular for arterial stiffening. As for its physiological role in vascular growth and maturation, we conclude that SSAO does not play a major role in cross-link formation of elastin or collagen in contrast to LOX, although it might provide a minor, accessory contribution. It appears probable that SSAO is involved in glucose transport and it may participate in VSMC differentiation, as it does in adipocytes, in which case it may modulate ECM protein production by VSMCs. It is clear that further studies are required to elucidate the exact role in vascular physiology of this enzyme which is highly expressed by VSMCs.
References
Cite this article
TY - JOUR AU - N. Mercier PY - 2009 DA - 2009/11/08 TI - The role of “semicarbazide-sensitive amine oxidase” in the arterial wall JO - Artery Research SP - 141 EP - 147 VL - 3 IS - 4 SN - 1876-4401 UR - https://doi.org/10.1016/j.artres.2009.10.002 DO - 10.1016/j.artres.2009.10.002 ID - Mercier2009 ER -