Spatial and temporal characteristics of Ca2+ signaling in endothelial cells of intact rat tail artery
- DOI
- 10.1016/j.artres.2013.03.002How to use a DOI?
- Keywords
- Spatial; Temporal; Calcium signaling; Endothelium; Carbachol; Culture
- Abstract
Very few studies have described briefly the spatial and temporal characteristics of Ca2+ signaling using ATP, bradykinin, histamine and thrombin in cultured endothelium. However so far, the spatial and temporal characteristics in intact endothelium have not been elucidated.
The aim of this work was to increase the understanding of characteristics of Ca2+ signaling in endothelial cells using real time confocal imaging of Fluo-4 loaded intact rat tail arteries. Of all the three agonist used i.e. histamine, bradykinin and carbachol; only CCh produced strong dose dependent stimulant action on the endothelial cells of intact rat tail artery. Local endothelial heterogeneity was observed in fresh intact cells in response to the three agonist used which was absent upon culture.
In agreement with the previous studies, each of the three agonists produced complex Ca2+ signaling consisting of initial fast followed by sustained component with Ca2+ oscillations superimposing it. The data supports the following conclusions: (i) Ca2+ puffs were evoked in 18% and 14% of cells by low concentrations of CCh (0.1 μM and 1 μM), respectively. The average diameter and width of Ca2+ puffs initiation sites was 1.9 ± 0.4 μm, 1.6 ± 0.1 μm, respectively. Length varied between 2 and 6 μm (n = 25 vessels). (ii) ECs responding with Ca2+ waves and oscillations were dependent on concentration of CCh: 0.1 μM (20%), 1 μM (65%) and 10 μM (99%). Speed of wave propagation and frequency of Ca2+ oscillations varied among different cells even at the same agonist concentration. (iii) Ca2+ waves were regenerative; initiated by one or both ends of the cell and propagated across the cell at a speed of 14–44 μm/s (iv) With increasing concentrations of CCh (0.1–10 μM), the frequency of Ca2+ oscillations ranged from 0.02 to 0.3 Hz with optimal frequency being about 0.2 ± 0.02 Hz (n = 15 vessels). In conclusions, this data shows the morphology, heterogeneity and detailed characteristics of Ca2+ signal in intact endothelial cells.
- Copyright
- © 2013 Association for Research into Arterial Structure and Physiology. Published by Elsevier B.V. All rights reserved.
- Open Access
- This is an open access article distributed under the CC BY-NC license.
Introduction & background
Vascular endothelium is characterized by heterogeneity both morphologically and functionally depending on the tissue in which they are located. This heterogeneity is displayed not only at different levels of vascular tree i.e. between macro and microvessels, but also cells isolated from different organs or different regions of the same organ.12 The endothelium of cerebral circulation is continuous, having tight junctions; endocrine glands and kidneys have fenestrated endothelium, where cells have intracellular and trans-cellular discontinuities.3,4,8 The use of cultured endothelial cells from different vascular beds and species14,21 strongly hampers our understanding of the different morphological and functional features observed at various parts of vascular system.
Due to technical limitations, much of the previous work has been done on isolated or cultured endothelium10,17 which may behave differently from intact vascular endothelium. Although the isolation and culture of EC has the advantage of investigating endothelial cell-specific processes, it is quite obvious that culture process might induce artifacts. Culturing EC may result indeed in the loss of specific functions and the introduction of new metabolic characteristics, which are not present in endothelial cells in vivo. These cultured endothelial cells compared to intact endothelium in situ may differ for both phenotypes and physiological characteristics resulting in modified responses. To the best of my knowledge no such study has been undertaken that explains the spatial and temporal aspects of Ca2+ signal in intact endothelium. Thus it is largely unknown, the temporal and spatial spread of Ca2+ signal in single or between cells in intact endothelium of large conduit arteries. In addition, comparison of analysis of [Ca2+]i variations in cultured and fresh endothelial cells of intact vessel, has not been investigated previously to assess homogenous or localized changes of [Ca2+]i to subgroups of endothelial cells. This study has addressed the hypothesis that fresh and cultured endothelial cells in situ display heterogenous Ca2+ signaling response to traditionally used agonist.
For this purpose, I have adopted in situ, simultaneous visualization of large numbers of EC with single cell resolution from intact rat tail artery endothelium. Using this approach I have observed in intact preparation, gross morphological changes when cultured in the presence of muscarinic and inflammatory agonists. The spatial and temporal characteristics of Ca2+ signaling varied between cells in fresh preparations. The local endothelial heterogeneity to CCh, histamine and bradykinin was very much pronounced which was absent upon culture.
Materials and methods
Tail was removed from rat which was humanely killed by cervical dislocation under CO2 anesthesia according to the University of Liverpool legislation requirements. Tail artery was dissected from the ventral grove, cleaned of fat and kept in physiological saline before use. Tissues samples (fresh & cultured) were loaded with Fluo-4 acetoxymethyl ester (Invitrogen, UK, 15 μmol/L; dissolved in DMSO with pluronic acid) for 2–3 h at 20 °C and then transferred to indicator-free solution for 30 min. All tissues were fixed to the bottom of the chamber by aluminum foil clips, to minimize movement. All experiments were performed at 30 °C. We used a Nipkow disc based, confocal microscope (Perkin Elmer), connected to a sensitive iXon cooled charge-coupled device camera (Andor). Images were collected at 30 frames per second using a ×60 water objective (NA 1.20) for best spatial resolution or dry (×20, 0.70 NA) for a larger field of view. Physiological saline of the following composition was used (mmol/L): NaCl 120.4, KCl 5.9, MgSO4 1.2, CaCl2 2.0, glucose 8, and HEPES 11. All chemical were purchased from Sigma (UK), except Fluo-4 AM and Pluronic F-127 which were from molecular probes (Invitogen). Stock solutions of CCh (10 mM), histamine (10 mM), bradykinin (20 mM), Caffeine (5 mM) and Cyclopiazonic acid (CPA, 20 μM) were prepared in distilled water. The maximal concentration of each of the agonists was first prepared in Kreb’s solution and through a series of dilutions different concentrations were obtained.
Culture
Samples of rat tail artery were dissected under sterile conditions, cleaned of fat and cut into strips of 4–5 mm at room temperature. Under sterile conditions, strips of rat tail artery were transferred to dishes containing 5 ml of culturing medium containing DMEM/Ham’s F12 1:1 plus 100 μl/ml of penicillin, 100 μg/ml of streptomycin, 5 ml of fetal calf serum and 500 μl of L-Glutamine. A maximum of 3–4 strips of rat tail artery were placed in each dish and put into water jacketed incubator at 37 °C and 5% CO2 in air. All chemical were purchased from Sigma, except DMEM from Invitrogen, UK.
Statistics
All analysis was performed using Microcal Origin 8.0 (Massachusetts, USA). Quantification of calcium records from confocal imaging were made by measuring the peak amplitude, frequency and duration of calcium transients. A minimum of 3–5 animals were used for each series of experiments. The results are given as percentage of control value unless otherwise stated. Values are given as means ± SEM, and n is number of vessels or cells.
Results
Live morphology of cultured and fresh endothelial cells
Confocal imaging showed 2-dimensional images in X–Y and Y–Z projections of the monolayer of the ECs of the rat tail artery which was obtained by taking a series of optical sections in z direction. ECs were flat, spindle shaped, lying in the groove of the basal lamina (Fig. 1A). The length of the ECs was 54 ± 1.7 μm and the width 6.5 ± 0.2 μm (n = 27 cells, 7 vessels). Figure 1B shows that ECs after 72 h of culture retained their morphology. Like fresh endothelium, cultured cells were spindle shaped, tightly packed with overlapping edges. The length of the cultured ECs was 55 ± 1.2 μm and the width 6.9 ± 0.5 μm (n = 31 cells, 5 vessels).

Live morphology of fresh and cultured endothelial cells loaded with Fluo-4. A: 2-Dimensional confocal images of intact ECs in X–Y (i), (ii) and Y–Z (iii) projections observed using ×60 objective. X–Y (i) & (ii) images show that ECs are spindle shaped, tightly packed with overlapping edges; Y–Z (iii) image shows that ECs are indeed present as a monolayer sitting in the grooves of the basal lamina. C, D, E: X–Y images of the cultured ECs in the presence of histamine (10 μM), bradykinin (1 μM) and carbachol (10 μM) after 72 h, loaded with Fluo-4 showing large inter-endothelial gaps, cells of irregular shapes producing thin long processes.
In order to characterize any changes in morphology of endothelial cell in the presence of histamine 10 μM, bradykinin 1 μM and carbachol 10 μM, the intact strips of rat tail artery were cultured for 3 days. We observed interendothelial gaps, cells of irregular shapes and size and thin long processes produced by individual ECs making contacts with several other cells in their vicinity as shown in Fig. 1C and D, E n = 5 vessels. It was not possible to measure the length or width of endothelial cells due to their bizarre shape.
Effects of carbachol, histamine and bradykinin on Ca2+ signaling of intact endothelial cells
Firstly, the responsiveness of intact endothelial cells to three different concentrations of CCh (0.1, 1, 10 μM), histamine (1, 10, 100 μM) and bradykinin (0.1, 0.5, 1 μM) were studied, using ×20 objective. This allowed observing Ca2+ events in a large number of cells in the field of observation. The results show that the maximal concentrations of bradykinin and histamine produced Ca2+ signaling only in small number of cells 7–8% n = 7 vessels while all cells in the field of observation were responding to CCh (Movie 1). In contrast, upon culture endothelial cells responded well to stimulation with bradykinin and histamine (Fig. 3) even with a minimal concentration used i.e. (0.1 & 1 μM) respectively. Despite the culture of intact endothelium, normal calcium signaling on application of CCh was observed.
Each of the agonists produced complex Ca2+ signaling consisting of initial fast followed by sustained component with Ca2+ oscillations superimposing it (Figs. 2 and 3). Since intact fresh endothelial cells were responding poorly to histamine and BK tested by the subsequent application of CCh, therefore in the later experiments CCh was used to investigate the spatial and temporal characteristics of Ca2+ signaling in intact endothelial cells. In preliminary experiments using ×20 objective I have found that the number of cells responding to CCh was dependent on the concentration of CCh (Fig. 2D). The response attained from 10 μM CCh was maximal and reproducible after every 5 min washout with kreb’s solution.
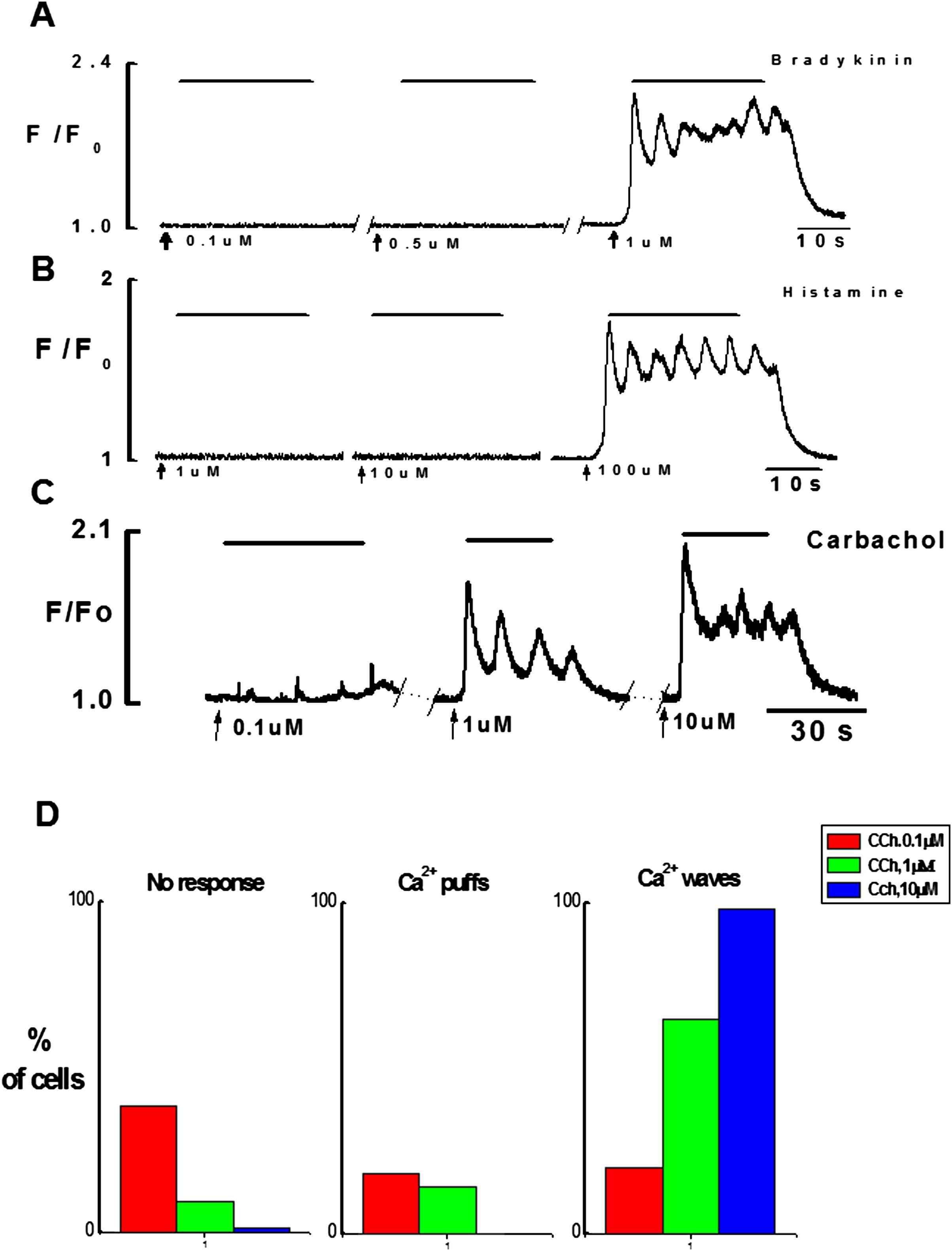
Effects of increasing concentrations of bradykinin, histamine and CCh on Ca2+ signaling in endothelial cells. A(i), B(i), C: Graphical records of Ca2+ transients induced by three different concentrations of bradykinin (0.1 μM, 0.5 μM & 1 μM), histamine (1 μM, 10 μM, 100 μM) and carbachol (0.1 μM, 1 μM, 10 μM). Ca2+ puffs were observed with low concentration of CCh (0.1 μM) however typical Ca2+ transient with maximal concentration of each agonist consists of initial phasic component followed by the sustained component superimposed by the Ca2+ oscillations. D: Bar chart shows percentage of endothelial cells responding to increasing concentrations of CCh.
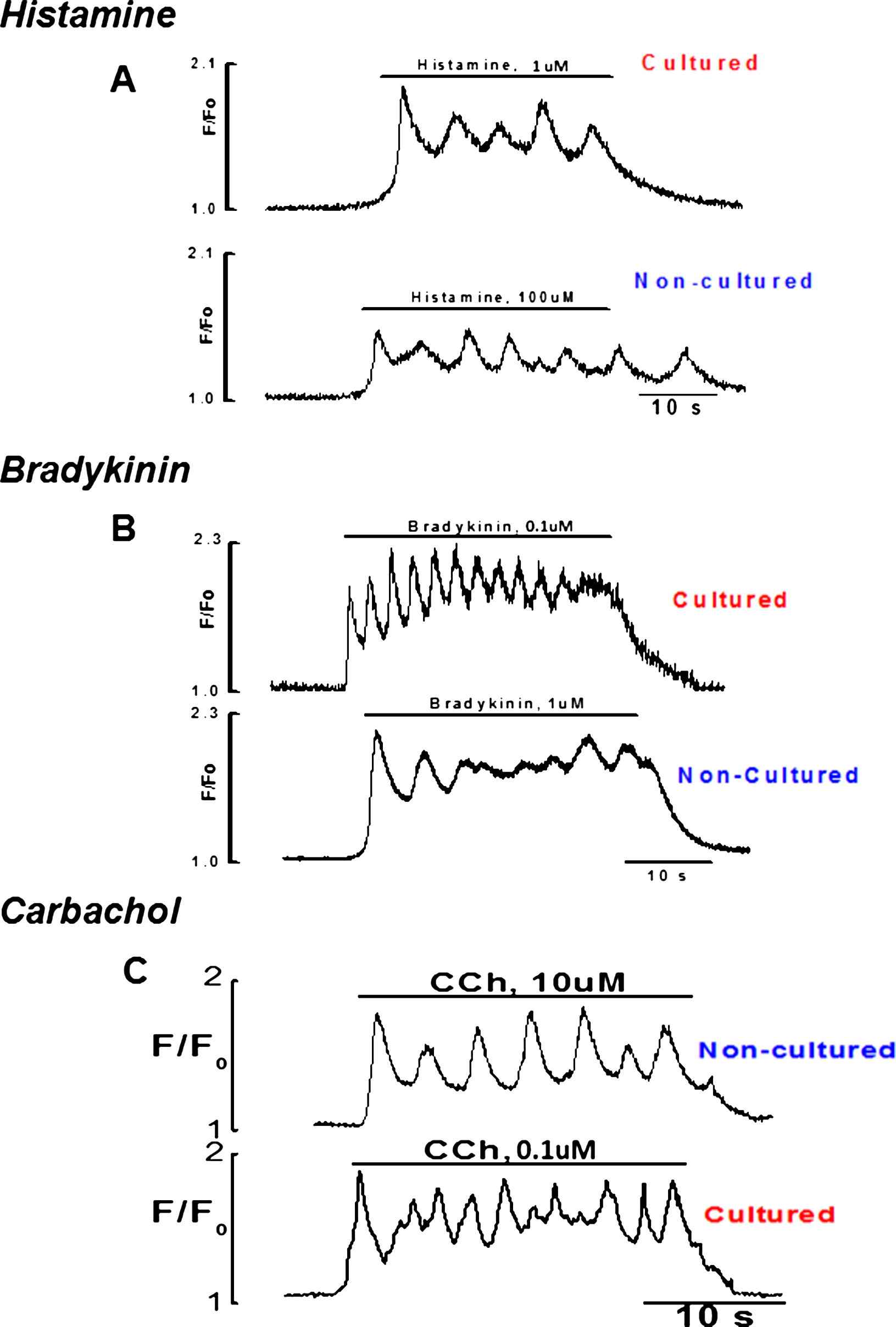
Graphical records of cultured and fresh endothelial cells to stimulation with minimal and maximal concentrations of histamine, bradykinin and CCh respectively.
Temporal and spatial characteristics of Ca2+ signaling in endothelial cells evoked by different concentration of CCh
Endothelial cells of the intact rat tail artery were not spontaneously active. However when stimulated by CCh, depending on concentrations some cells responded with localized Ca2+ events i.e. Ca2+ puffs and some with global Ca2+ events i.e. Ca2+ waves/Ca2+ oscillations. Figure 2D shows that the number of cells which did not respond to CCh was: 38% for 0.1 μM, 9% for 1 μM and only 1% did not respond to 10 μM of CCh. Ca2+ puffs were evoked in 18% and 14% of cells when stimulated by low concentrations of CCh (0.1 μM and 1 μM, respectively). Ca2+ waves and oscillations were evoked by all concentrations of CCh. However, the number of cells responding with global Ca2+ signaling was dependent on concentration of CCh: 0.1 μM (20% of cells), 1 μM (65% of cells) and 10 μM (99% of cells).
To demonstrate the homogeneity of Ca2+ release in response to non-agonist mediated triggers, addition of CPA (20 μM) to Ca2+ free solution produced a transient elevation of intracellular Ca2+ caused by Ca2+ leak from the ER (Fig. 4). After application of CPA for 20 min in Ca2+ free solution the ER was fully depleted which was tested by the lack of any response to the application of CCh. Addition of 2 mM Ca2+ caused large sustained rise of [Ca2+]i. Subsequent application of CCh(10 μM) produced a typical Ca2+ transient.
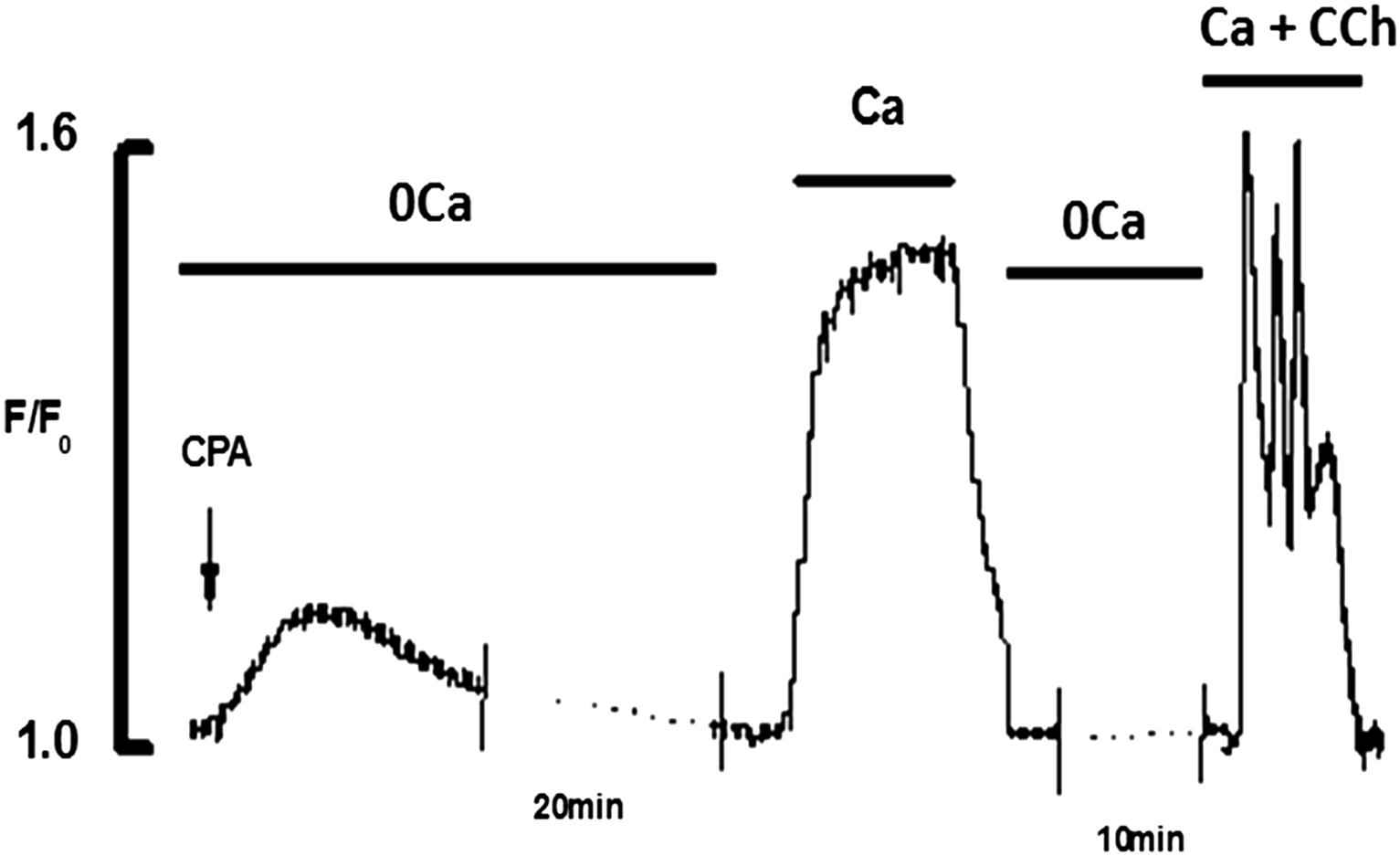
Effects of addition of Ca2+(2 mM) and CCh(10 μM) to CPA(20 μM) depleted ECs. CPA in 0Ca2+ produced transient elevation of [Ca2+]i and fully depleted the store in 20 min. Addition of 2 mM Ca2+ produced a large rise of [Ca2+]i. Addition of CCh in the presence of Ca2+ produced the typical Ca2+ transient.
Ca2+ puffs
Ca2+ puffs induced by low concentrations i.e. 0.1 and 1 μM of CCh, originated from one or two discharging sites located at the ends of the endothelial cells (18 cells) and main part of the body (4 cells). The initiation sites located at the ends of the cells were elongated (8/13) and those located in the perinuclear region were circular (5/13) in shape. The average diameter of the circular sites was 1.9 ± 0.4 μm. The width of the elongated sites was 1.6 ± 0.1 μm and the length varied between 2 and 6 μm (n = 13cells, 3 vessels). Ca2+ puffs were non-propagative and recorded only in the discharging site. They were not detected several microns away from the discharging site (Fig. 5A and B). Typical examples of Ca2+ puffs triggered by 0.1 μM CCh in several endothelial cells of same preparation are shown in Fig. 5 and movie 2. The amplitude, duration and frequency of Ca2+ puffs showed large variability not only among different cells (Fig. 5C) but also for different sites of the same cell.
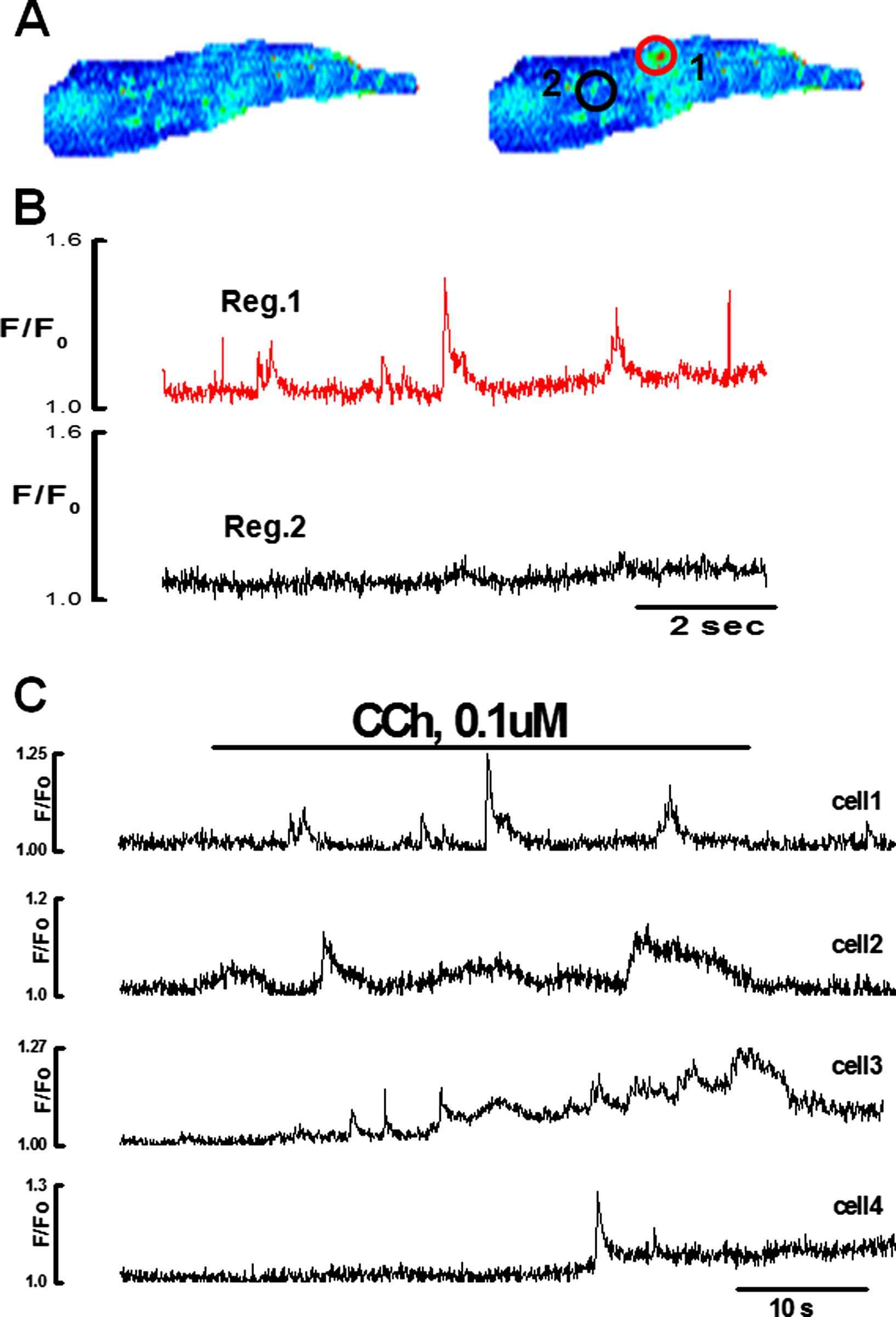
Ca2+ puffs induced by the low concentration of CCh in the intact endothelial cell n = 9. A:X–Y pseudo color images of endothelial cell in the absence (i) and the presence of 0.1 μM CCh showing one active discharging site located in the perinuclear region of the cell. B: Graph showing Ca2+ puffs in region 1. No activity recorded in the region 2 C: Patterns of the Ca2+ puffs evoked by 0.1 μM CCh recorded from discharging sites of the four different endothelial cells showing a large variability in the amplitude, duration and frequency among different cells.(For interpretation of the references to colour in this figure legend, the reader is referred to the web version of this article.)
Functional testing of Ca2+ puffs and transient was done by using a putative activator of the ryanodine receptor channels caffeine (2–5 mM) and 2-APB. In these experiments ryanodine had no effect on CCh induced Ca2+ puffs or transient (Fig. 6). However, 2-APB (50 μM) abolished CCh induced Ca2+ puffs and transient. The data suggest that the ECs of the rat tail artery do not have functional RyRs channels and indeed IP3Rs are responsible for the observed Ca2+ events.

Effect of caffeine (5 mM) and 2-APB (50 μM) on Ca2+ puffs and transient induced by 0.1 & 10 μM CCh, n = 8 respectively. Application of caffeine or ryanodine had no effect on Ca2+ signal in endothelial cells; however, 2APB for 30 min blocked the Ca2+ puffs and transient.
Ca2+ waves
Ca2+ transient induce by CCh in the ECs was complex and consisted of the initial fast component followed by sustained component superimposed by Ca2+ oscillations both in fresh and cultured endothelial cells (Figs. 2, 3 and 7). The initial component always appeared as a propagating Ca2+ wave (Movie 3). Ca2+ waves were regenerative and were normally initiated by one or both ends of the cell and propagated across the cell at a speed of 14–44 μm/s (n = 20 cells, 4 vessels) in different directions (Movies 3 and 4). There was no difference observed in the speed of wave propagation in cultured endothelium. Ca2+ wave was preceded by slow rise of Ca2+ (called pacemaker) which upon reaching a threshold produced a fast upstroke. Average value for time to peak of the initial phasic component was 1.24 ± 0.2 s (n = 20 cells, 4 vessels).
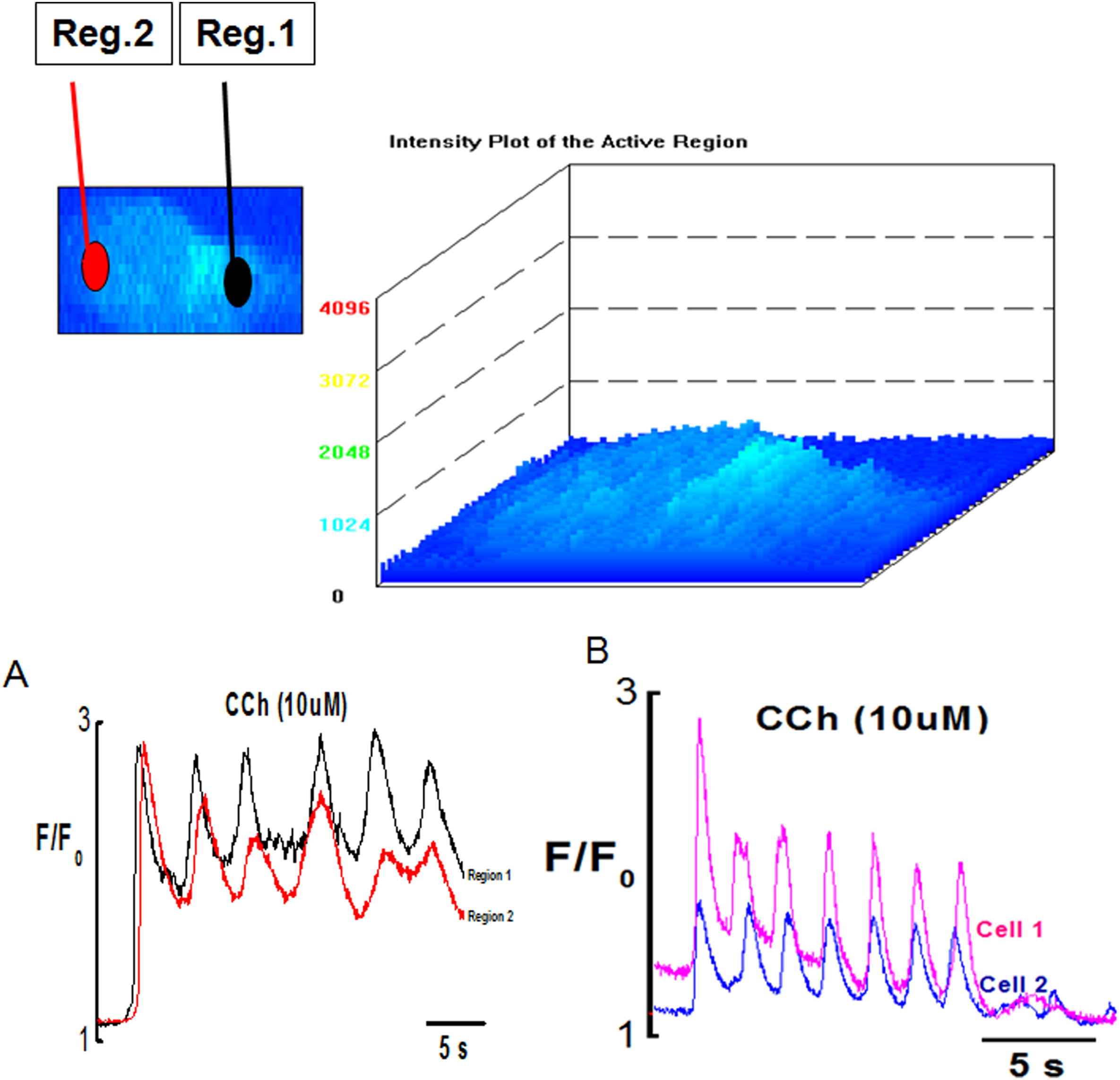
Surface plot of Ca2+ oscillations in endothelial cells induced by CCh (10 μM). (A) Graph showing synchronized Ca2+ wave and oscillations in Reg.1 and Reg. 2 of the endothelial cell (B) synchronized Ca2+ waves and oscillations between two different cells of same preparation.
Ca2+ oscillations
Intracellular Ca2+ oscillations which normally followed the initial Ca2+ wave were observed in majority of the ECs (Fig. 7 & Movie 5). Poor or no oscillations were seen in cells stimulated with low dose (0.1 μM) of CCh with the frequency of Ca2+ oscillations ranging from 0.02 to 0.08 Hz with an optimal frequency being about 0.05 Hz (n = 20 cells, 4 vessels). Frequency of Ca2+ oscillation increased with higher concentrations of CCh. In the presence of 10 μM CCh the frequency of Ca2+ oscillations ranged from 0.08 to 0.3 Hz with optimal frequency being about 0.2 Hz at room temperature n = 63cells, 4 vessels. Ca2+ oscillations were asynchronous among different cells showing variations in amplitude, frequency and duration at lower agonist concentration (0.1 μM). However, the synchronicity of Ca2+ oscillations produced within a single cell and between cells was dose dependent. At higher agonist concentration (1 μM, 10 μM), the oscillations produced within and between different EC’s became synchronized as shown in Fig. 7A and B, and Movie 5.
Discussion
An elevation of [Ca2+]i in endothelial cells plays an important role in the regulation of agonist stimulation. There is complexity in [Ca2+]i kinetics. This study has described a novel method that can be used for characterization of calcium signal in intact endothelium from rat tail artery. The study has shown changes in calcium signal in response to different agonist stimulation in fresh and cultured intact endothelium; local and global Ca2+ events with reference to temporal and spatial characteristics.
Technical advantages
The main advantage of this method was that it eliminated completely possible effects of collagenases or trypsin while cells remained in their in situ position. There was no tissue manipulation and tissue was quickly obtained. This method enabled to study large number of endothelial cells from each specimen in a short period of time. The employed technique allowed to study interactions between the components of the vessel wall.
Ca2+ signaling
The experiments conducted demonstrate that the response and pattern of Ca2+ signaling in fresh endothelial cells differs profoundly from that in the cultured cells. With intact fresh endothelial cells in situ, the majority responded to CCh in the observed field. On the other hand 6–7% of the cells in the observed field responded to bradykinin and histamine. Interestingly, I have also noted that the cells responded poorly to bradykinin and histamine and subsequent application of CCh in poorly responding cells produced a maximal response. This observation is in agreement to previous study of15 which suggested entirely heterogenous population of cells in rat aorta. In this study, population of cells responding to different agonist was observed; however, due to receptor diversity, a set population of cells responded either to one or more than one agonist. The receptors on a set of cell population can either be up or downregulated depending on a particular physiological or a pathological state. We observed a multitude of Ca2+ signaling patterns, such as Ca2+ puffs, Ca2+ oscillations and well-maintained plateau phases, which reflected activation of Ca2+ entry mechanisms.
Upon culture, the heterogeneity of Ca2+ signaling in response to CCh, histamine and bradykinin was lost. All cells in the field of observation responded equally and maximally to each of the agonist used. This was a very important observation which discourages the use of cultured endothelial cells for research purposes. Similar observations were made by26 when comparing freshly isolated and cultured bovine endothelial cells. The point to ponder is that cultured endothelial cells should be used cautiously and carefully. Further studies are required to identify changes at the biochemical level in culture condition. This might explain the heterogenous observations made while investigating the identity of store operated channels in cultured endothelial cells from various sources.20,28,29
Spatial and temporal Characteristics of Calcium signaling in intact endothelium
Our experiments show that the pattern of Ca2+ signaling varied profoundly from cell to cell. The processes were more sensitive to agonist stimulation. They were the preferential initiation sites for Ca2+ signaling in intact endothelial cells of rat tail artery. The intracellular Ca2+ rise and rate of wave propagation were always faster at the initiation sites either at the processes or in the cell body. This higher sensitivity of initiation sites could be due to the aggregation of Ca2+ release channels, presence of more sensitive Ca2+ release channels or the presence of modulatory factors that increase IP3 sensitivity such as Ca2+.6,5
The intracellular Ca2+ in endothelial cells could produce Ca2+ puffs only or spread as a wave to the rest of the cell depending on channels recruitment. Ca2+ puffs were recorded only in the discharging site stimulated with low CCh concentration suggesting very limited Ca2+ diffusion. This limited diffusion could be due to strong buffering as seen in other cell types.30,1 Propagating Ca2+ wave therefore require the co-ordinated release of Ca2+ from the store. Diffusion characteristics of Ca2+ or IP3 and agonist concentration are responsible for this temporal delay that reflects variation in speed of wave propagation in different cells as has been suggested in previous studies.2,18,25
The speed of wave propagation increased with increase in agonist concentration. Similar findings were observed in other cell types.11,13,16,19 Calcium wave was regenerative and involved IP3 receptors7,9,27 Pretreatment of endothelial cells for 30 min in ryanodine (50 μM) had little or no effect on CCh induced Ca2+ puffs and Ca2+ transient suggesting the absence of functional ryanodine receptors (Fig. 6).
Sustained component of Ca2+ transient was superimposed by Ca2+ oscillations. The frequency of Ca2+ oscillations was variable and concentration dependent; increased frequency with increase in agonist concentration seen in other cell types.22–24 It has been shown that these Ca2+ oscillations are produced as a result of interplay between Ca2+ release and reuptake by the ER and Ca2+ influx through CCE channels18 however the focus of the present study is on heterogenous responses observed between cultured and fresh endothelium by using three agonist with detailed spatial and temporal Ca2+ signal characteristics.
Conclusions
In conclusion, this paper has shown that the response and pattern of Ca2+ signaling in fresh endothelial cells differs profoundly from that in the cultured cells. Fresh endothelium showed heterogeneity of Ca2+ signaling in response to CCh, histamine and bradykinin which was not the case in cultured ECs. So the use of cultured endothelial cell should be discouraged. This study also showed that the initiation sites were more sensitive to agonist stimulation independent of their location, a faster intracellular Ca2+ rise and increased rate of wave propagation than the rest of the cell. Generation of Ca2+ wave and oscillation were dependent on the agonist concentration. This variability of Ca2+ signaling could have important physiological and pathological implications.
Conflict of interest
The author do not have any conflict of interest.
Acknowledgments
This work was supported by
References
Cite this article
TY - JOUR AU - Sadaf Mumtaz PY - 2013 DA - 2013/03/28 TI - Spatial and temporal characteristics of Ca2+ signaling in endothelial cells of intact rat tail artery JO - Artery Research SP - 84 EP - 92 VL - 7 IS - 2 SN - 1876-4401 UR - https://doi.org/10.1016/j.artres.2013.03.002 DO - 10.1016/j.artres.2013.03.002 ID - Mumtaz2013 ER -