Vascular adaptation to extreme conditions: The role of hypoxia
- DOI
- 10.1016/j.artres.2016.02.003How to use a DOI?
- Keywords
- Hypoxia; High altitude; Endothelial function; Arterial stiffness; Carotid remodeling
- Abstract
The study of vascular adaptation to extreme conditions, and in particular to hypoxia, represents a unique opportunity in cardiovascular physiology, with relevant translational implications. First, it has crucial clinical consequences for about 140 million people worldwide living at high altitude and chronically exposed to hypobaric hypoxia. Second, an increasing number of lowlanders are exposed to high altitude for recreational or working purposes, including aged, diseased individuals: in these cases, hypoxia could be a trigger for acute cardiovascular events. Finally, hypoxia plays a major role in the pathogenesis of many diseases and chronic conditions, as respiratory (i.e. chronic obstructive pulmonary disease and obstructive sleep apnea syndrome) and cardiovascular disorders (i.e. heart failure, ischemic heart disease and cerebrovascular disease). Thus, results from field studies at high altitude might be important for a deeper understanding of their pathophysiology. This review is aimed at summarizing the main findings in the field of chronic and acute vascular adaptation to hypoxia, focusing on the role of nitric oxide (NO) and endothelial function, as well as large artery behavior.
- Copyright
- © 2016 Association for Research into Arterial Structure and Physiology. Published by Elsevier B.V. All rights reserved.
- Open Access
- This is an open access article distributed under the CC BY-NC license.
Introduction
During human evolution, several homeostatic mechanisms, involving the cardiovascular, respiratory and neuroendocrine system, have been developed in order to maintain stable tissue O2 levels. NO is a key molecule in systemic and pulmonary vascular physiology, for its vasodilating, antithrombotic and antimitotic properties.1 Reduced NO availability in the systemic circulation, which is the main feature of endothelial dysfunction, has been recognized as the first step towards development of atherosclerosis.1 During exposure to hypoxia, NO is crucial to ensure O2 and nutrients delivery to tissues and it is considered the main responsible for early hypoxic vasodilation. For example, NO metabolites such as nitrite are supposed to be converted again to NO under hypoxic conditions and may induce vasodilation both by endothelium-dependent and -independent pathways.2 An increased expression of several NO synthase (NOS) isoforms has also been demonstrated.3 The balance between reactive oxygen species (ROS) and NO pathway seems also to be crucial for successful vascular adaptation to hypoxia, since increased ROS production is the main cause of NO destruction. Indeed, low O2 concentrations may be a cause of overproduction of ROS together with decreased levels of antioxidant capacity, leading to oxidative damage to lipids, proteins, and DNA, and finally to cellular death.4 Under a more prolonged exposure to hypoxia, other regulatory mechanisms take place. After few hours-days, hypoxia-induced peripheral vasoconstriction, sympathetically mediated as a consequence of chemoreflex activation, prevails on direct vasodilation,5 leading to increased blood pressure.6 Sympathetic activation is also able to induce endothelial dysfunction,7 while oxidative stress per se is also able to induce sympathetic activation,8 thus inducing a vicious circle. A late effect of high altitude acclimatization is an increased proliferation of red blood cells, aimed at achieving a greater oxygen-carrying capacity to overcome the low ambient oxygen tension. Subsequent hyperviscosity might also influence negatively cardiovascular homeostasis and vascular function. The key for successful adaptation to hypoxia probably resides in the complex balance between these contrasting forces. In this framework, the study of individuals and populations naturally predisposed to successful acclimation to high altitude, a powerful challenge to the cardiovascular system triggering mechanisms underlying most chronic non-communicable diseases,9 might suggest novel strategies and therapeutic targets for cardiovascular disease.
Acute adaptation to hypoxia
A number of vascular abnormalities were reported after acute exposure to normobaric hypoxia10,11 and high altitude, including impairment of endothelial function and arterial stiffening6,12–15 As explained above, among mechanisms hypothesized, hypoxia-induced ROS production, sympathetic and endothelin system activation are supposed to play a relevant role.13,16 On the other hand, exposure to 2–4-h simulated hypobaric hypoxia did not modify flow-mediated dilation17 or reactive hyperemia index18 in young healthy individuals. In a recent study by our group, endothelial dysfunction after 4-h hypobaric hypoxia was present only in individuals prone to develop acute mountain sickness, but not in the asymptomatic ones.19 An overview of the main characteristics and findings of the studies dealing with this topic is in Table 1. A high degree of inhomogeneity emerges between different studies both in the techniques used and districts studied for the exploration of vascular function and structure, in the ascent protocol and in the study timeline, making it difficult to draw firm conclusions. However, beyond methodological issues, some physio-pathological aspects are worth special consideration.
Study | Technique | Exposure | Timeline | Study population | Main results |
---|---|---|---|---|---|
Bakker E et al., 201541 | FMD | Khumbu Valley, Nepal (3700–4200 m) by 7-day trekking | 1 and 3 days | 10 healthy lowlanders | FMD reduced |
Bruno RM et al., 201419 | FMD, PWV, carotid geometry and stiffness | Aiguille du Midi, France (3842 m) by cable car | 4 h | 22 AMS−, 12 AMS+ healthy lowlanders | FMD unchanged in AMS−, reduced in AMS+; PWV unchanged; cIMT reduced, carotid diameter increased; carotid elasticity increased in AMS- |
Lewis NC et al., 201413 | FMD, PWV, carotid geometry and stiffness | Ev-K2-CNR Pyramid Laboratory, Nepal (5050 m) by 9–11-day trekking | 3–4 days and 12–14 days | 12 healthy lowlanders | FMD reduced; PWV increased; cIMT reduced, carotid diameter increased |
Parati G et al., 20136 | PWV, Aix (Applanation tonometry) | Capanna Regina Margherita, Monte Rosa, Italy (4559 m) by cable-car + 1-day trekking) | 24–72 h | 42 healthy lowlanders | PWV unchanged, AIx increased |
Rhodes HL et al., 201114 | SI and RI by finger photoplethysmography | 3450 m and 4770 m | 11 days | 11 lowlanders (3 hypertensives) | SI unchanged, RI reduced |
Frick M et al., 200615 | FMD | Obertauern, Austria (1700 m) by bus | 1 and 21 days | 18 individuals with metabolic syndrome | FMD unchanged after 1 day, reduced after 21 days |
Iglesias D et al., 201517 | FMD | Simulated hypobaric hypoxia (hypobaric chamber, equivalent to 4000 m) | 4 h | 10 healthy lowlanders | FMD unchanged |
Johansson PI et al., 201418 | RHI by peripheral arterial tonometry | Simulated hypobaric hypoxia (hypobaric chamber, equivalent to 4500 m) | 2–4 h | 12 healthy lowlanders | RHI unchanged |
Vedam H et al., 200942 | Aix, TR (Applanation tonometry) | Normobaric hypoxia (SO2 80%) | 20 min | 12 healthy lowlanders | AIx reduced, TR unchanged |
Frobert O et al. 200843 | FMD | Normobaric hypoxia (FIO2 = 0.12) | – | 10 healthy lowlanders + 10 individuals with CV risk factors | FMD reduced |
Thomson AJ et al. 200622 | AIx (Applanation tonometry) | Normobaric hypoxia (SO2 80%) | 1 h | 8 healthy lowlanders | AIx reduced |
FMD: flow-mediated dilation; PWV: pulse wave velocity; cIMT: carotid intima-media thickness: AIx: Augmentation Index; TR: time to reflection; CV cardiovascular; RHI: Reactive hyperemia index; SI: stiffness index; RI: reflectivity index.
Summary of studies about systemic vascular function and structure after acute exposure to hypoxia.
A major variable to be taken into account is concomitant physical exercise. Comparing studies conducted with same technique and standardized methodology for the assessment of endothelial function,13,15,17,19 an impaired endothelial function is evident only in studies in which high altitude was reached by exerting a prolonged physical effort, which might be associated per se with increased levels of circulating biomarkers of inflammation and endothelial activation.20 However, there is also the intriguing possibility that the great inter-individual differences that have been reported in acute vascular response to hypoxia might represent a key factor in determining successful adaptation. In the Resamont-2 study we tested the hypothesis that vascular response to hypoxia is early impaired in individuals who will develop acute mountain sickness (AMS) after 24 h-hypobaric hypoxia. For this purpose, we recruited 34 apparently healthy individuals, born and living at sea level. Endothelial function, aortic and arterial stiffness were evaluated in Aosta, Italy 583 m.s.l. and 4 h after passive ascent by cable car to 3842 m.s.l. in Aiguille du Midi, France. The volunteers were re-evaluated for AMS occurrence after 24 h at 3842 m.s.l. Individuals who developed AMS had a greater SO2 worsening and FMD reduction after 4-h hypobaric hypoxia, well before symptoms onset.19 Conversely, FMD was unchanged in asymptomatic individuals, and accompanied by a significant increase in shear rate area under the curve. Based on these results, we hypothesized that in lowlanders with successful adaptation to hypoxia, maintenance of preserved flow-mediated dilation is conceivably obtained through an increased post-ischemic response in the microcirculation, probably attributable to a favorable balance between hypoxia-induced vasoconstrictor and vasodilator pathways, as described in the introduction. This adaptive mechanism seems to be less efficient in individuals susceptible to AMS. In keeping with our findings, lower levels of exhaled NO have been associated with AMS occurrence in a Chinese population sample.21 The hypothesis of systemic vascular maladaptation to hypoxia as a key mechanism for high altitude-related diseases is supported by the findings of Berger and coauthors, who reported an impaired endothelial function in the microcirculation of lowlanders susceptible to high altitude pulmonary edema, but not in the asymptomatic ones, after exposure to acute normobaric hypoxia.10
Large artery behavior after exposure to high altitude is also a mostly unexplored topic. To date, the studies conducted suggest that aortic stiffness, measured as pulse wave velocity (PWV) is unchanged in lowlanders after acute exposure to high altitude,6,19 while is steadily increased after 3–4 days at high altitude.13 It is conceivable that only a prolonged and sustained exposure to high altitude is able to alter this parameter. Conversely, relevant changes in carotid mechanics and hemodynamics are evident after only 4 h of exposure to high altitude, highlighting also a different behavior between individuals prone or resistant to AMS.19 In the Resamont-2 study, though carotid enlargement was observed in both groups, it was associated with an increase in carotid elasticity and in peak systolic velocity in the mean cerebral artery in only in individuals not developing AMS. These results confirm that in the first hours of exposure to high altitude, vasodilatation, particularly in the cerebral district, may be an early adaptive response, aimed at maintaining cerebral oxygenation despite hypoxemia.6,22 Our findings, showing dilatation accompanied by increased elasticity, suggest a direct vasodilatory effect of hypoxia on smooth muscle cells in the carotid wall, which explains also the reduction in wave reflection evident in our study.19 This vasodilatory response to hypobaric hypoxia is early blunted in AMS+ individuals, well before symptoms onset, thus suggesting a pathogenic role.
This hypothesis is supported by the demonstration of a beneficial effect of acetazolamide in preventing AMS symptoms as well as BP and wave reflection changes.6 This protective effect may depend on its action on vascular responsiveness to chemoreflex-mediated sympathetic activation rather than to changes in central or peripheral chemoreflex sensitivity,23 since acute intravenous administration of acetazolamide induced an increase in velocities in the middle cerebral artery at high altitude, possibly due to an increased NO generation.24
Taken together, the aforementioned evidence supports the hypothesis that systemic vascular maladaptation to hypoxia might be responsible of AMS development. However other hypotheses should be taken into account. Studies performed in experimental animals suggest that, at least for high-altitude cerebral edema, an altered permeability of the blood–brain barrier, rather than hemodynamic changes in the cerebral vasculature, might play a relevant role.25 Indeed, inflammatory molecules and reactive O2 species might induce both blood–brain barrier leakage and systemic endothelial function, leading to misinterpretation of study results. However it is important to notice that the only study conducted in humans on this topic demonstrated that altered cerebral hemodynamic regulation and increased oxidative stress occur in healthy volunteers developing AMS, while no signs of blood–brain barrier dysfunction were present.26
Chronic adaptation to hypoxia
Many residents of the Himalayan valleys and Tibetan Plateau live at high altitude, experiencing O2 concentrations that are about 40% lower than those at sea level. Compared to other populations living at high altitude, namely the Andean populations, they developed a favorable phenotype, characterized by lower prevalence of pulmonary hypertension and polycythemia, despite decreased arterial O2 content.27
This favorable phenotype is related to a distinct set of genomic variants characterizing this population and mostly involving hypoxia-inducible factor (HIF) pathway genes,28 transcription factors that induce the transcription of hundreds of genes involved in maintaining oxygen levels in tissues.29 Interestingly, neuronal and endothelial isoforms of NOS are also HIF targets, in line with a number of observations demonstrating an increased NO generation in this population.30 This mechanism might explain the reduced incidence of elevated pulmonary artery pressure and chronic mountain sickness (CMS) in Himalayan natives.
As far as the systemic circulation is concerned, a compensative vasodilation, which has been attributed to tonically elevated NO production,27,30 has been demonstrated in the microcirculatory district in Himalayan natives.10,31 Our group recently demonstrated that compensative vasodilation occurs even in large arteries: indeed carotid diameter was significantly greater in Nepalese high-altitude dwellers in comparison to sea-level Caucasian healthy volunteers.32 Interestingly, enlarged diameter, whose possible causes were already discussed, was accompanied by reduced IMT, leading to reduced W/L and increased circumferential wall stress, as confirmed also by Lewis and coauthors.13 Several interpretations are possible for this finding: a feature of smooth muscle relaxation but also an expression of maladaptive remodeling, in parallel to the findings in chronic kidney disease patients.33
Despite the acute vascular effects of hypoxia, as explained above, are still a matter of debate, chronic exposure to hypoxia is invariably associated with impaired endothelial function (Table 2). This finding was confirmed in healthy Nepalese high-altitude dwellers,13,32 in Aymara high-altitude dwellers with chronic mountain sickness34 as well as in patients with obstructive sleep apnea syndrome.35 While the findings in Aymara individuals with chronic mountain sickness were expected, given the presence of profound hypoxemia, erythrocytosis and reduced NO generation,27 the causes of endothelial dysfunction in Himalayan populations are still unknown (Fig. 1). Noteworthy, reduced hyperemic stimulus was a relevant, independent determinant of FMD in this population,32 at variance to what observed in healthy Caucasian volunteers.36 It is conceivable that the peculiar genetic background in Himalayan dwellers, protecting them from polycythemia and pulmonary hypertension, can negatively influence stimulated NO release, or that it is simply not sufficient to offset chronic effects of hypoxia in the systemic circulation. Whatever the cause, the presence of endothelial dysfunction in Himalayan high altitude dwellers appears to be of clinical relevance, since even in this healthy population, without any classical cardiovascular risk factor, a lower FMD is related to increased left ventricular mass and carotid IMT, which are established surrogate endpoints of cardiovascular events. Thus, impaired endothelial function might be associated with increased cardiovascular risk also in this population. Although to date the prevalence of cardiovascular risk factors and disease in this area is unknown, ischemic heart disease and diabetes represent the first cause of death in developing countries, with their health burden increasing over years.37
Study | Technique | Exposure | Study population | Main results |
---|---|---|---|---|
Bruno RM et al., 201432 | FMD, PWV, carotid geometry and stiffness | Khumbu Valley, Nepal (2600, 3800 and 3800 m) | 95 healthy Nepalese highlanders | FMD reduced; PWV unchanged; reduced cIMT, increased carotid diameter |
Lewis NC et al., 201413 | FMD, PWV, carotid geometry and stiffness | Ev-K2-CNR Pyramid Laboratory, Nepal (5050 m) | 10 healthy Nepalese highlanders | FMD reduced; PWV increased; cIMT reduced, carotid diameter increased |
Rimoldi SF et al., 201234 | FMD, PWV, cIMT | La Paz, Bolivia (3600 m) | 23 Aymara patients with chronic mountain sickness | FMD reduced; PWV increased; cIMT increased. |
Otsuka K et al., 200544 | CAVI | Ladakh, India (3524 m) | 19 Ladhaki elderly highlanders | CAVI unchanged |
FMD: flow-mediated dilation; PWV: pulse wave velocity; cIMT: carotid intima-media thickness: CAVI: Cardio-Ankle Vascular Index.
Summary of studies about systemic vascular function and structure in populations chronically exposed to hypoxia.
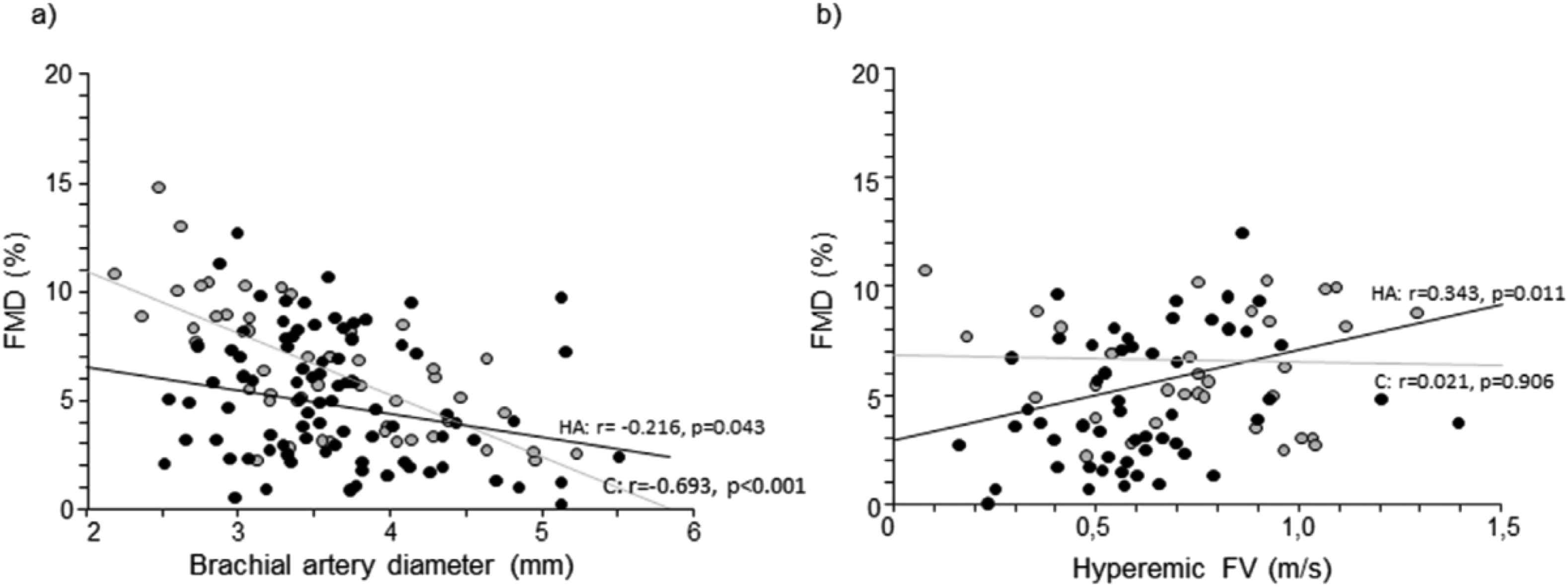
Scatter plots representing the relationship of flow-mediated dilation (FMD) with brachial artery diameter (a) and hyperemic flow velocity (FV) (b) in Caucasian sea-level controls (C, gray circles) and Himalayan high-altitude individuals (HA, black circles). From Bruno et al.,32 with permission.
Perspectives and future directions for research
Vascular physiology at high altitude is a complex research field, influenced by several regulatory pathways with different impact over time: thus, future studies should include serial multi-parametric evaluations in order to achieve a better comprehension of the timing of the phenomena. Furthermore, an accurate standardization of techniques used and of ascent protocols is mandatory. The role of confounding factors such as blood viscosity, hydration, nutrition patterns, socio-economic status should be ascertained, especially for studies regarding native Andean, Himalayan or Ethiopian populations. Genotype-phenotype correlation studies, for different patterns of acute and chronic systemic vascular adaptation to hypoxia, are still lacking and they could help identify mechanisms beyond different routes of adaptation. In particular, no information are available on vascular function and structure of the inhabitants of the Ethiopian plateau, which are likely to exhibit a totally different pattern of genetic variants.38 The metabolic aspects, such the role of insulin resistance,39 are still to be explored among mechanisms underlying vascular dysfunction at high altitude. The clinical consequences of individual vascular response to hypoxia in high-altitude dwellers and in lowlanders exposed to high altitude for working or recreational reasons are an exciting and promising field of research. Finally, exposure to hypoxia might constitute a sort of “stress test” for the cardiovascular system, possibly unmasking predisposition to cardiovascular disease at an early stage, in parallel to the results obtained for pulmonary vascular function.40
Conflict of interest
The authors have no conflicts of interest to disclose.
Acknowledgments
The research was founded by the SHARE Project (Stations at High Altitude for Research on the Environment – Ev K2 CNR) and by the
References
Cite this article
TY - JOUR AU - Rosa Maria Bruno AU - Lorenzo Ghiadoni AU - Lorenza Pratali PY - 2016 DA - 2016/02/22 TI - Vascular adaptation to extreme conditions: The role of hypoxia JO - Artery Research SP - 15 EP - 21 VL - 14 IS - C SN - 1876-4401 UR - https://doi.org/10.1016/j.artres.2016.02.003 DO - 10.1016/j.artres.2016.02.003 ID - Bruno2016 ER -